A Quick Guide to Editing The Competence And Contexts, 2e
Below you can get an idea about how to edit and complete a Competence And Contexts, 2e step by step. Get started now.
- Push the“Get Form” Button below . Here you would be taken into a splashboard allowing you to make edits on the document.
- Choose a tool you want from the toolbar that appears in the dashboard.
- After editing, double check and press the button Download.
- Don't hesistate to contact us via [email protected] for any questions.
The Most Powerful Tool to Edit and Complete The Competence And Contexts, 2e
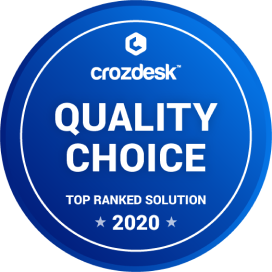
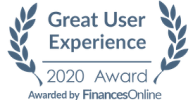
A Simple Manual to Edit Competence And Contexts, 2e Online
Are you seeking to edit forms online? CocoDoc can help you with its powerful PDF toolset. You can utilize it simply by opening any web brower. The whole process is easy and quick. Check below to find out
- go to the free PDF Editor page.
- Upload a document you want to edit by clicking Choose File or simply dragging or dropping.
- Conduct the desired edits on your document with the toolbar on the top of the dashboard.
- Download the file once it is finalized .
Steps in Editing Competence And Contexts, 2e on Windows
It's to find a default application capable of making edits to a PDF document. Luckily CocoDoc has come to your rescue. Take a look at the Manual below to find out how to edit PDF on your Windows system.
- Begin by adding CocoDoc application into your PC.
- Upload your PDF in the dashboard and make edits on it with the toolbar listed above
- After double checking, download or save the document.
- There area also many other methods to edit PDF files, you can go to this post
A Quick Manual in Editing a Competence And Contexts, 2e on Mac
Thinking about how to edit PDF documents with your Mac? CocoDoc has the perfect solution for you. It enables you to edit documents in multiple ways. Get started now
- Install CocoDoc onto your Mac device or go to the CocoDoc website with a Mac browser. Select PDF document from your Mac device. You can do so by hitting the tab Choose File, or by dropping or dragging. Edit the PDF document in the new dashboard which includes a full set of PDF tools. Save the file by downloading.
A Complete Manual in Editing Competence And Contexts, 2e on G Suite
Intergating G Suite with PDF services is marvellous progess in technology, with the potential to chop off your PDF editing process, making it quicker and more cost-effective. Make use of CocoDoc's G Suite integration now.
Editing PDF on G Suite is as easy as it can be
- Visit Google WorkPlace Marketplace and find CocoDoc
- install the CocoDoc add-on into your Google account. Now you are ready to edit documents.
- Select a file desired by clicking the tab Choose File and start editing.
- After making all necessary edits, download it into your device.
PDF Editor FAQ
Can you explain a challenging concept at two different levels?
The Concept of Chemical Bonding and the Octet Rule.LEVEL 1:Most atoms just want to be happy, just like you.In chemistry a happy atom is an atom with a full atomic shell.I will keep this very simple and explain it using an analogy with chickens.Suppose you are a chicken and you have a number of eggs. In our hypothetical chicken world you have the nestlings and the normal chickens.The nestlings are so small that they can handle up to 2 eggs.The grown up chickens can hold up to 8 eggs.Some really big chickens can hold even more eggs, but I promised to keep it simple so for the sake of argument let's stick with “2-egg nestlings” and the normal “8-egg chickens”)Now we introduce the concept of a Happy chicken.A Happy chicken is a chicken with just 8 eggs not 9, not 7; just 8. (Obviously the baby chickens are happy with 2 eggs.)Some chickens have too many eggs let’s say one or two extra. Some other chickens are really close to their ideal egg-state and have only a few short to the ideal egg-number (i.e. 2 or 8).Those chicken would go around looking for other chickens that want to give up an egg or two.Now the fun starts. You have different strengths in chickens. Depending how strong each chicken is compared to the other chicken they will take the egg and run away. We call those ionic eggs.But sometimes the chickens are almost the same strength. In that case even if each chicken tries to pull and run away with an egg, it will not happen: the other chicken is just too strong.If it were humans this would end up with a continuous fight in order to take control over the eggs, but our hypothetical chickens are smart animals they came up with a simple solution: they just share the eggs.They both keep responsibility for their common eggs. (Those eggs are called covalent eggs.)Eggs can be shared with one other chicken, but sometimes chickens make a real chicken community and eggs are shared with multiple chickens. They can make single shared eggs, but sometimes they share 2 or even 3 eggs with each other.In the end, each chicken just wants to be a happy chicken and they all want to have 8 (or 2) eggs they do not really care if they need to share them.You might ask how we will know that some chickens share eggs and some not. There is no good answer to that question, but a really important rooster of the chicken world (aka Rooster Linus Pauling) measured the strength of all chicken families. He called this the egg-negativity scale.Based on this scale you can calculate the difference of their egg-negativities, and so you will know if the eggs is shared or not. Sometimes the eggs are nicely in the middle, but there are many cases where the eggs are actually almost entirely ‘owned’ by the one of the chickens. But that's ok chickens just want to be happy.There is one particular special chicken family: Carbon chickens. They normally have just 4 eggs. Since they need 4 extra eggs to be happy chickens and almost no other chicken family wants to give away that much of their eggs, the carbon chickens learned to be really good in sharing eggs with other carbon chickens. In fact they are really good at sharing eggs with almost any other chicken family.The carbon chickens are the most flexible chickens in the world!So to conclude: Happy Chickens.(Pictures come from 1 and 2)LEVEL 2: (under)graduate levelIonic bonding is the complete transfer of valence electrons between atoms.Covalent bonding is the sharing of valence electrons between atomsMetallic Bonding sharing of free electrons in a lattice structure of (positively charged) cations.The difference between an ionic and covalent bonding is often explained in terms of electronegativity.I’m not going to repeat the full history and explanation. See e.g. electronegativity.In summary: Linus Pauling was the first to put electronegativity in a quantitative scale he described it as “the power of an atom in a molecule to attract electrons to itself.”I just want briefly discuss some aspects that are still taught to students and - in my opinion- do more harm than good.In an early chemistry education they often use an arbitrary value of 1.7 as minimum difference in electronegativity in order to have an ionic bond. Obviously that’s an oversimplification.In this context you will often find the so-called van Arkel Ketelaar bond-type triangles. I added a revised version adapted from Jenssen (J. Chem. Educ. 395-398, 72, 1995)Source: van Arkel-Ketelaar TrianglesIt visuales the progressive transition from idealized metallic, covalent, and ionic bonding. The main thing students should remember from this triangle is that it's a continuum most chemicals are neither 100% covalent nor 100% ionic.The value of 1.7 mentioned above actually corresponds to an estimation from Pauling himself: a difference greater than 1.7 would give a bond which is predominantly ionic.Or using the formula of Pauling to calculate the ionic character[math](1-e^{-(\Delta \chi/2)^2} )\times 100\%[/math][math]\Delta \chi[/math] is the difference in electronegativity: a value of 1.7 gives 51.4%I hope you agree that setting the boundary at 50% is rather arbitrary.If I talk to early chemistry students I almost never hear that critical nuance. But they are not to blame: the concept is not explained correctly.OCTET configurationI do not want to discuss quantum mechanics. So I’m just going to postulate the octet rule as a rule of thumb.The idea that atoms bond with each other in order to achieve octets because they want to be 'stable' or 'happy' (sic) is obviously an incorrect or vague description. (I’m not a biologist, although I would agree that animals — like chickens — can be happy, elements do not have feelings.)A chemical bond is the result of the summed attractive and repulsive electrostatic interactions between a number of positively charged nuclei and a number of negatively charged electrons.If we would look to Sodium Chloride the simplistic explanation would be that the chloride ion and sodium cation are formed in order to achieve octet configuration. But in reality this will only happen in solution the main reason would be via interactions with the solvent.Solid NaCl does not have discrete ions: it forms a giant crystal lattice.The problem with the octet rule starts when students are using this rule to construct some molecules based on Lewis-dot structures: many text books only cover the very simple covalent bonding between two atoms. The so called 2c–2e bonding (2 centres, 2 electrons bonding) and they often use incorrect explanations to explain what is going on.Take a very simple molecule: the sulfate-ion.Let’s use a section from a random linkFour distribution of bonds are shown below with the formal charges of all the atoms. These structures differ only in the number of double bonds on the central sulfur atom.In the absence of any other type of information, we would choose structure C because it has the lower set of formal charges. We would choose structure C rather than structure D (which has the same set of formal charges) because it is unlikely that a negative charge would reside on sulfur, which is less electronegative than oxygen.You might notice that the structure we have chosen is hypervalent, with more than an octet of electrons around the central atom. This is acceptable because the sulfur d-orbitals can participate in the bonding.I could have chosen other: see e.g. link, or Sulfate - Wikipedia, but I bet that 95% of the chemistry students learned that structure C is the ‘correct’ way to represent the sulfate ion. The sulfur has two double bonds and therefore has 12 valence electrons instead of the ‘normal’ octet, but “Sulfur is using its d-orbitals’ so that’s ok.”What if I would tell you that Structure A is probably the most predominant representation of the sulfate ion? Indeed it’s fully compliant with the octet rule, it does not need any hypervalent explanation (or —for heaven's sake — d-orbitals) and my statement is backed up with multiple articles around the subject: indeed using quantum mechanical calculations about 68% of true structure can be represented using normal octet configuration (so structure A !!) . (23% can be represented by 12 equivalent structures; 1.9% each) [1]Note: the structure with the oxygen completely detached is just a representation. (It might not be a good idea to include that in earlier courses of chemistry!)Also in other relatively simple molecules like PF5 they are still using traditional 2c-2e bonding and when you would draw Lewis dot structures of that molecule you will get something like:Phosphor has 10 electrons instead of 8.But they just tell students: ‘P is able to use its d-orbitals, so that’s ok’Indeed according to Valence Shell electron Pair Resulsion Model (VSEPR theory) electrons are promoted to the empty d-orbitalsThey are supposed to form 5 sp3d hybrid orbitals and this explains the formation of a trigonal bipyramid.Again just one small problem: quantum mechanical calculations show that the d-orbitals are not necessary.In order to explain this type of structures adequately you could include other types of bonding in this case a 3c-4e bond (3 center, 4 electron).Equatorial: 3 sp2 ‘normal’ hybrid orbitals (a 2c–2e bond)Axial: Overlap of the unhybridized p orbital of P (containing 2 electrons) and two orbitals of Fluor containing one electron each giving a 3c–4e bondScheme CHEM241 Course Notes - 3I know these type of bonds are a bit controversial, but a similar type of bond can be used to explain electron deficient species like BF3: a 3 center 2 electron bond (or 3c-2e).In addition I’m not claiming that you need to explain this type of bonding in early chemistry courses:I do understand that a theory is just a theory and in most cases you start with simple models that needs to be revised or dealt in more depth in subsequent years. But in my opinion it is not a good idea to discuss a theory that is wrong or in the words of the author of this article [ref 2]Although the idea of spd hybridization is simple enough for general chemistry students to grasp and it works well to describe the bonding in hypervalent molecules, it is fundamentally flawed and therefore should be removed from the general chemistry curriculum.So my advice would be just keep it simple: the concept of a Lewis structure is a very good educational tool. But make it absolutely clear that it has its downsides. In addition — in early chemistry classes — I would certainly avoid hypervalent or electron deficient molecules; there is plenty of time to explain those in later years.Further reading[1 ] On the Role of d Orbital Hybridization in the Chemistry Curriculum[2 ] Common Textbook and Teaching Misrepresentations of Lewis Structures[3] How relevant are S=O and P=O Double Bonds for the Description of the Acid Molecules H2SO3, H2SO4, and H3PO4, respectively?[4] Lost in Lewis Structures: An Investigation of Student Difficulties in Developing Representational Competence[5] Applications of spin-coupled valence bond theoryThis is a more or less a combination of two of my early answers.Kurt Van den Broeck's answer to Why is there PCl5 but no NCl5?Kurt Van den Broeck's answer to Why is a covalent bond formed by organic compounds?It was fun reusing the ‘chicken and egg’ analogy although I do realise that every simplification has it's own down-side.As side note I also realise that chemistry is not that popular on Quora: I really do not know why, so I’m not sure if this answer has any value. But If one chemistry teacher reads it (Dave?, Abeeha?) and uses some of it in his or her classes, I would be a really ‘happy’ person.
What are the irrational numbers between 3 and 6?
Since we are talking about irrational numbers and ordering, set R is the set in context.Fact about irrational number is that you can find an irrational number between any two irrational number.Another simple statement is that average of an international and rational number is irrational.Let a be rational and b be irrational. Define c as (a+b)/2. So, 2c=a+b. So, 2c-a=b. If c is rational then 2c-a is rational. So, b becomes rational. A contradiction. So, c must be irrational.Now our task in simple.3^2 is 9 and 6^2 is 36. Take numbers which are not compete squares (for example, 10, 11, 12, 13, 14, 15, 17, …, 35; 10 to 35 except 16 and 25) and square roots of them.All these are irrational numbers; these are algebraic irrationals.e is approximately 2.73 and transcendental irrational. 2e is irrational and between 3 and 6.Pi is approximately 3.14. again transcendental and between 3 and 6.We got two required numbers. If we go pn taking averages iteratively, we can get infinitely many desired numbers.
Why do they still teach the chemiosmotic theory of oxidative phosphorylation (proton gradient and all that) when this peer-reviewed paper proves it is impossible?
Well, he offers (on page 6) six reasons why it is impossible, but I don’t think any of them stand up to scrutiny. I will address them individually. This is going to be a bit long, but I think it is important.1. (The main point) mitochondrial matrix lacks adequate protons for any "outward pumping" kind of activity.(<10^1, at the physiological pH) in a mitochondrion-Yes, because the mitochondrion is small, if you calculate the number of protons at a reasonable pH the number is quite small. But this is like saying, since electrolysis of water reduces H+ to hydrogen gas, and there are only 10^-7 moles of H+ per liter, we can only get 0.5×10^-7 moles of H2 from a liter of water. He is forgetting that water dissociates, H2O is a very good H+ donor. Proton transfer reactions are very fast, diffusion-limited, reactions. Also, until the mechanisms are nailed down, it could just as well be transport of OH- into the mitochondrion as transport of H+ out: the two are not experimentally distinguishable. In either case, as the internal pH goes up, the OH- concentration is increasing more than the H+ concentration is decreasing.Two of the strongly supported proton uptake mechanisms are through the reduction of O2 to 2H2O and reduction of quinone Q to QH2. If the oxidase reduces O to make O-2 and surround it with water at any pH reachable by the matrix, it will pick up a proton from H20 giving two OH-. At this point you have deposited 2 OH- in the matrix per 2e-: one from the O-2 that got protonated and one from deprotonation of water. Likewise if you reduce Q to Q-2 it will rapidly be protonated to QH2, because the pKa's are above and about 10. (More likely the protons are transferred from amino acids H-bonding the substrate, concomitantly with electron transfer, and the amino acids reprotonate after the product dissociates). There is no problem of running out of protons in the matrix, what you are really doing is building up the concentration of hydroxide. And you will reach a pH of 9 and a very powerful delta-pH of 2 while deprotonating less than 1/10^5 of the water molecules.(Apparently all this has been explained to the author, he dismisses it with: "Lysis of water's O-H bond or . . . cannot be sources either". Really? Why not?)2. “the system cannot work in steady state because the function of thousands of molecular pumps cannot be synchronized. . . .”There is no need for synchronization- the reactions can take place randomly at different times. The proton gradient is like a capacitor that charges up with outward translocation of many protons, and discharges as they are taken back in by ATP synthase. During steady state phosphorylation, there would be a relatively constant proton gradient as pumps add to it one (or two) protons at a time, and the synthases takes away from it one proton at a time. No "temporally staggered potential development and dissipation" of the gradient is envisioned!There are big excesses of NAD+/NADH, ubiquinone, and to a lesser extent cytochrome c, compared to the respiratory chains (~20 quinones per complex III). These provide a "pool" function, buffering between their reducers and their oxidizers. If they are getting reduced faster than oxidized, then the pool becomes highly reduced, but this slows down the reducers and speeds up the oxidizers so that a steady state is reached where both rates are equal. The "pools" supply the substrates for each complex, with no need to synchronize with other complexes.The Synthase can take one proton at a time from the gradient, moving forward 1/8 of a turn with each (and sometimes moving backwards 1/8 turn and returning a proton to the gradient). As it turns a torque builds up, inhibiting further forward motion. It is believed that the stator arms and perhaps the rotor have a good deal of flexibility, acting like springs so the c-ring can turn and build up torque. The torque is applied to the catalytic head group and when the nucleotide binding sites are in the proper occupancy the head group will turn 1/3 turn, producing 1 ATP and releasing the torque. No need for synchronous delivery of protons, or nucleotides or phosphate.3. “The chemiosmosis hypothesis violates fundamental laws of thermodynamics by assuming that a dissipated force/energy is recycled through the same modality. That is, protons moving in and out of the same membrane in a closed system cannot give a viable machine for doing useful work.”Not sure what he is saying here- the transmembrane proton gradient is like a capacitor or battery that is continuously being charged up by a generator while supplying power to an electric motor or other device. It works for the Prius!4. “Advocates of chemiosmosis insist that mOxPhos yields only 2.5 and 1.5 molecules of ATP per molecule of NADH and succinate respectively (the so-called P/O value)33. This is when the cellular metabolic requirements clearly solicit significantly higher efficiencies.24In addition, the fact is that markedly higher yield (3.5 and 2.2 molecules of ATP per molecule of NADH and succinate respectively) have been consistently reported by many reputed groups since the 1940s and emphatically asserted even in the last few decades.”The reasons for variability in reported values of P/O have been described by Hinkle (1). There are more than one way of measuring the ratio, and each requires certain assumptions and corrections to be made. Hinkle's "meta-analysis" concludes that intrinsic ratios of around 2.5 and 1.5 are most consistent with all the results. (Now that the stoichiometry of the synthase is known(2) we can revise that up slightly to 2.72 and 1.63 theoretical maxima.)For example, when measuring the amount of oxygen taken up during phosphorylation of a defined amount of added ADP, there is already significant oxygen consumption before adding the ADP ("state 4 rate"). Do you assume that rate continues in parallel with the oxygen uptake due to phosphorylation, and so subtract the state 4 rate from the state 3 rate? The state 4 rate is attributed to proton leaks, which occur when it is all charged up with no ADP to phosphorylate. It is now known that the leak is exponentially related to the magnitude of proton gradient. Once ADP is added, phosphorylation partially dissipates the proton gradient and the leak becomes insignificant. If you subtracted out the state 4 rate you seriously underestimated the oxygen consumption, thus overestimating the P/O ratio.5. ”Furthermore, the simple/non-modularized mitochondrial architecture and membrane proteins' random distribution (and structure of proteins per se) therein do not support the operational feasibility of proton pumps or/and chemiosmosis-based build-up of TMP. Quite simply, it is highly unlikely that a few protons' movement could provide the torque for Fo module's rotation within the membrane/F1 subunit. In this context, it is inappropriate to compare the flagellar motor (which has a very different molecular assembly) that is supposed to make a rotation using thousands of protons”.Assuming a membrane potential plus pH gradient equivalent to 22 kJ/mol, 8 protons supplies 176 kJ/mol of free energy. The deltaG for ATP synthesis is around 40 kJ/mol.(Exact value depends on ATP/ADP and phosphate and Mg++ concentration). So there is no problem for 8 protons to produce 3 ATP. As for supplying torque to spin the rotor, Brownian motion is already doing that for free, the proton gradient and transport mechanism just provide strong enough directionality to keep it going mainly in the direction of ATP synthersis.6. “Table 1 presents a compilation of 2e input via complex I or II. If proton pumps worked, Complexes I, III, and IV could pump a maximum of three, one, and five protons, respectively (or a total of only nine protons) per one NADH molecule\u2019s oxidation. The corresponding RCPE consensus values are as follows: 4, 4/2, and 2/4. This quantitative disparity means that the explanation in textbooks are not thoroughly researched and/or corroborated.In addition, this means that proton accounting is totally misplaced. (The original set of explanations posits that four protons each were pumped out through the Complexes I, III, and IV; giving a total of 12 protons per 2e-traverse through ETC [from NADH]. Earlier, these 12 protons were fully required for performing one complete rotation of Complex V [to give three ATP molecules], which made some structural sense because the c drum was comprised of 12 subunits. Such assumptions were "conveniently changed by an authoritative consensus" [as mentioned in point (4) above] to 10 protons (with only three protons needed for 120° movement or synthesis of an ATP molecule by Complex V]. To arrive at the number 10, the earlier consensus was Complex III pumped only two protons, whereas Complexes I and IV pumped four each. Then, it was conveniently changed to Complexes I and III pumped four protons, whereas Complex IV pumped only two.)”-Not authoritative consensus, but rather new structural knowledge: The structure of yeast Fo c-ring (the first mitochondrial structure obtained) had 10 subunits(2) implying 10 protons per revolution, and it was assumed this held for all mitochondrial ATP synthases. However since 2005 it has been known that the bovine (and presumbaly other vertebrate) synthase has 8 subunits(3).Otherwise the author has done a pretty good job in Table 1 of summarizing current knowledge of the stoichiometry of the respiratory chain, except for some understandable confusion about whether Complex III pumps 4 protons per 2e- and IV pumps 2, or vice versa. The answer (explained below* to avoid getting side-tracked here) is that energetically speaking, with the mitochondrial (inside N-side) orientation, C_III is pumping two protons and C_IV is pumping 4 (per 2e-).With that cleared up we can take the values the author uses with 2H+ for CIII and 4 H+ for CIV. For redox energy available at the three "coupling sites", he calculates 69.5, 36.7, and 112 kj/(2 mol e-) going from NADH to ubiquinone, UQ to cytochrome c, and cytc to oxygen; based on the midpoint reduction potentials of the redox couples involved. Assuming these steps pump 4, 2, and 4 H+/2e-, against a proton gradient of 22 kJ/mol, the required free energy is 88, 44, and 88 kj/mol. Plenty of energy at the last step, but the first two come up a bit short.But this large proton gradient (22 kj/mol) is measured under state 4 conditions, where electron transport is stalled by the proton gradient because there is no ADP to be phosphorylated. The proton gradient builds up, with nothing but leaks to release it, and respiration slows down because it is pumping protons against a bigger and bigger gradient. The electron transport chain becomes the bottleneck, so under these conditions the NADH pool is highly reduced (by the added substrate or,in vivo, by the TCA cycle) and cyt c is highly oxidized.The free energy available depends not only on the standard redox potential but also on the ratio of oxidized to reduced forms, as described by the Nernst equation. If NADH is 99% reduced, that makes its potential 60 mv more negative (n=2 couple), adding about 12 kJ/mol to the energy available per 2e- for the first step and bringing the reaction close to equilibrium: 87.5 vs 88 kJ/mol. If cyt c is 99% oxidized, that raises its potential by 120 mv (n=1 couple), adding 24 kJ to the energy per 2e- available for the second reaction. This makes it quite spontaneous (72.7 vs 44) so it will continue until the Q/QH2 couple is somewhat oxidized, and that will get the first step going by adding to the energy from NADH to Q. So approaching equilibrium (except for the cytochrome oxidase reaction which is essentially irreversible), but that is what we expect under these conditions. Nothing is dissipating the proton gradient, so it builds up until it approaches equilibrium with the redox energy, and respiration slows to a crawl. This is the explanation of the respiratory control ratio.Now when ADP is added and phosphorylation begins to dissipate the proton gradient, the ET reactions become highly spontaneous and respiration speeds up. Now the bottlenecks are kinetic not thermodynamic, and we do not have the extreme red/ox ratios that we used above. But now the proton gradient is smaller, and we do not need that extra energy. Still no problem for the chemiosmotic model.To make this clearer we can make a table suggesting the conditions at each step assuming either state 4 or state 3 conditions and the resulting redox energy available for each step. Under state 4 we propose extreme red/ox ratios, meaning that one form or the other is at low concentration and thus not "kinetically competent" for ATP synthesis at maximum rate- this is OK because there is no ADP to be phosphorylated under these conditions. For state 3 we will assume the red/ox ratios are close to 1/1, both forms are present at high concentration allowing very fast proton pumping. Under these conditions the energy available at each step will be lower, but but the proton gradient is lower due to rapid depletion by the synthase. And that lower proton gradient is still powerful enough to phosphorylate ATP because the phosphate potential is lower.substrate NADH/NAD+ UQH2/UQ cyt_c(R)/cyt_c(O) H20/O2* Em -320 +50 +240 +820 state 3 red/ox 1/1 1/1 1/1 - Eh -320mv +50 +240 +820mv kj/2mol \-- 74 ---/ \--- 38 ----/ \-- 116 --/ Needed for 4,2,4: 60 30 60 (assuming ∆µH+=15 kj/mol) state 4 red/ox 100/1 1/5 1/20 - Eh -410mv 113 357 +820 mv kj/2mol \---94----/ \----45-----/ \---100---/ Needed for 4,2,4: 88 44 88 (assuming ∆µH+ =22 kj/mol) Here I am using the Faraday F = 100 instead of 96 for convenience so that kJ/mol = mv/10. If there is interest I will come back and do it right, but this won’t change much.(*The Eh of the H2O/O2 couple is not important because in all cases it is high enough to drive the oxidase reaction- for practical purposes this reaction is irreversible) (Actually value used is for 1 Atm (760 tor) O2. In mitochondria in working muscle it is more like 20 tor. but this 32-fold difference would only lower the potential of the O2/H20 couple by 34 mv, since it is an n=4 reaction.)[edit 2019 06] Apparently I was using 90 mv instead of 60 (59) mv for the change in effective potential for a 10-fold change in red/ox ratio. I have corrected that now and the values are closer in state 4 but still compatible with quasi-equilibriun between electron transport and a 220 mv proton gradient.[/edit]*Does Complex III pump 2 protons and Complex IV pump 4, or vice versa?In any case, together they pump 6. In fact Complex III releases 4 protons on the cytoplasmic (P) side, and takes up 2 on the matrix side. Complex IV releases 2 protons P-side and takes up 4 from the matrix. The extra protons are attributed to what Mitchell called "scalar" protons- oxidation of Quinol releases two protons, and reduction of 1/2 O2 to water consumes 2 protons.In terms of electrical charge there is no problem: in Complex III two e- cross the membrane for every two going to cyt c, and in complex IV two electrons cross the membrane to reduce O2 with protons from the N side while two more protons are "pumped" for 4 charges. But for the pH, i.e. concentration gradient, it depends on on which side the pH changes. In mitochondria, the cytoplasm is at relatively constant pH, and the matrix goes alkaline on energization. So in mitochondria the energetics of release of 4 protons outside is independent of the pH gradient, while the uptake of two protons inside is made less spontaneous by the high pH. So energetically Complex III is pumping 2 protons against the pH gradient in mitochondria. For experiments in inverted submitochondrial particles the situation is reversed, because the interior gets acid and that acidity opposes the release of 4 protons inside, so Complex III is pumping 4 protons against the pH gradient. This is discussed in ref 4, where they were using submitochondrial particles and looking at the equilibrium of cyt c with the succinate/fumarate couple in the presence of different ratios of ATP/ADP, to determine the H/2e stoichiometry of Complex III. They found it made a difference whether the Proton Gradient consisted mainly of ∆ψ or ∆pH; the latter being more effective at rev electron transport through complex III.References:1. Peter C. Hinkle (2005) Biochimica et Biophysica Acta 1706 (2005) 1-11 P/O ratios of mitochondrial oxidative phosphorylation.2. Stock D1, Leslie AG, Walker JE. Molecular architecture of the rotary motor in ATP synthase. Science. 1999 Nov 26;286(5445):1700-5. Molecular architecture of the rotary motor in ATP synthase.3. Watt et al. (2010) Bioenergetic cost of making an adenosine triphosphate molecule in animal mitochondria.4. Scholes TA, Hinkle PC. Energetics of ATP-driven reverse electron transfer from cytochrome c to fumarate and from succinate to NAD in submitochondrial particles.
- Home >
- Catalog >
- Miscellaneous >
- Manual Sample >
- Parts List Sample >
- Competence And Contexts, 2e