A Step-by-Step Guide to Editing The Analysis Of Biomolecular And Cellular Systems 2011 Problem Set 1
Below you can get an idea about how to edit and complete a Analysis Of Biomolecular And Cellular Systems 2011 Problem Set 1 in detail. Get started now.
- Push the“Get Form” Button below . Here you would be taken into a dashboard allowing you to conduct edits on the document.
- Pick a tool you need from the toolbar that pops up in the dashboard.
- After editing, double check and press the button Download.
- Don't hesistate to contact us via [email protected] for any help.
The Most Powerful Tool to Edit and Complete The Analysis Of Biomolecular And Cellular Systems 2011 Problem Set 1
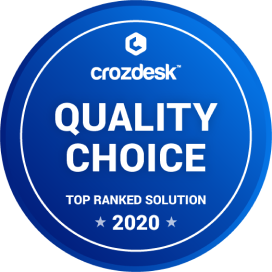
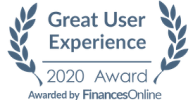
Complete Your Analysis Of Biomolecular And Cellular Systems 2011 Problem Set 1 Right Away
Get FormA Simple Manual to Edit Analysis Of Biomolecular And Cellular Systems 2011 Problem Set 1 Online
Are you seeking to edit forms online? CocoDoc has got you covered with its comprehensive PDF toolset. You can quickly put it to use simply by opening any web brower. The whole process is easy and quick. Check below to find out
- go to the PDF Editor Page.
- Drag or drop a document you want to edit by clicking Choose File or simply dragging or dropping.
- Conduct the desired edits on your document with the toolbar on the top of the dashboard.
- Download the file once it is finalized .
Steps in Editing Analysis Of Biomolecular And Cellular Systems 2011 Problem Set 1 on Windows
It's to find a default application capable of making edits to a PDF document. Fortunately CocoDoc has come to your rescue. Take a look at the Manual below to form some basic understanding about how to edit PDF on your Windows system.
- Begin by adding CocoDoc application into your PC.
- Drag or drop your PDF in the dashboard and make edits on it with the toolbar listed above
- After double checking, download or save the document.
- There area also many other methods to edit PDF online for free, you can check this guide
A Step-by-Step Guide in Editing a Analysis Of Biomolecular And Cellular Systems 2011 Problem Set 1 on Mac
Thinking about how to edit PDF documents with your Mac? CocoDoc offers a wonderful solution for you.. It empowers you to edit documents in multiple ways. Get started now
- Install CocoDoc onto your Mac device or go to the CocoDoc website with a Mac browser. Select PDF file from your Mac device. You can do so by pressing the tab Choose File, or by dropping or dragging. Edit the PDF document in the new dashboard which provides a full set of PDF tools. Save the paper by downloading.
A Complete Guide in Editing Analysis Of Biomolecular And Cellular Systems 2011 Problem Set 1 on G Suite
Intergating G Suite with PDF services is marvellous progess in technology, with the potential to simplify your PDF editing process, making it troublefree and more cost-effective. Make use of CocoDoc's G Suite integration now.
Editing PDF on G Suite is as easy as it can be
- Visit Google WorkPlace Marketplace and search for CocoDoc
- set up the CocoDoc add-on into your Google account. Now you can edit documents.
- Select a file desired by hitting the tab Choose File and start editing.
- After making all necessary edits, download it into your device.
PDF Editor FAQ
What is the recent advancement in mass spectrometry?
New mass spectrometry (MS) methods, collectively known as data independent analysis and hyper reaction monitoring, have recently emerged. These methods hold promises to address the shortcomings of data-dependent analysis and selected reaction monitoring (SRM) employed in shotgun and targeted proteomics, respectively.Proteomic strategies can be divided into two approaches according to their purpose (Fig. 1). One is peptide mass fingerprinting (PMF) by MALDI-MS, which is used for detailed protein identification in a high-throughput manner (Lam, Li, Lo, Guggenheim, & To, 2007). In this method, it is important to isolate proteins as much as possible using two-dimensional electrophoresis (2D-PAGE). The other is shotgun proteomics, which is used for global peptide identification by hybrid MS (precursor ion selection, MS1, and then product ion detection, MS2). In the shotgun method, it is necessary to first digest the protein mixture, and then accurately separate the large number of generated peptides using high-performance LC (Stephanowitz, Lange, Lang, Freund, & Krause, 2012). From the viewpoint of protein separation, 2D-PAGE is one of the best protein separation protocols. However, this method has issues such as a complicated procedure and poor reproducibility. Immobilized pH gradient gels and automated 2D-PAGE systems have been developed, but these still have not lead to solutions for intrinsic recovery. Currently, the first choice for proteomic approaches is usually LC-ESI-MS, because of improvements in nanoliter-flow LC, column resins, probe performance, automated MS and MS/MS analysis, and the basic potential for MS and its peripheral devices (Howells et al., 2005, Iwasaki et al., 2010, Sun et al., 2014). In addition, MS is a platform that analyzes molecules after they are ionized into the gas phase. Therefore, the molecules must be ionized in some way, and the ionization technologies, fast atom bombardment (FAB) (Morris, Panico, Karplus, Lloyd, & Riniker, 1982), electron ionization (EI), chemical ionization (CI) (Oka et al., 1991), and specific chemical modification are available (Calderon-Celis, Encinar, & Sanz-Medel, 2017). MALDI and ESI are widely used, especially for biomolecules (Hommerson, Khan, de Jong, & Somsen, 2011). These ionization reactions are called “soft ionization” methods. Since the precursor ions of the biomolecule are usually not cleaved, structure-derived product ions from the biomolecule are easily detected (Standing, 2003).Fig. 1Fig. 1. Two proteomic approaches by shotgun LC-MS/MS analysis and 2D-PAGE/PMF.Proteins are extracted correctly from biological samples. 1) Proteins are first digested by trypsin protease after denaturation and reductive alkylation. And generated peptides are separated by reverse-phase HPLC, and directly induced into ESI interface. MS and MS/MS spectra are obtained by data-dependent acquisition. 2) Proteins are denatured in reductive chaotropic solution with non-ionic detergent, and separated by isoelectric point and molecular weight. Each protein spots are digested by trypsin after reductive alkylation. PMF spectra are obtained after purification and mixing with ionization matrix. Protein identification is performed by proteome database server by using processed MS/MS and PMF spectra for the determination of post-translational modification or molecular annotation.Additionally, the amount of detected ions can also be measured. Considering the feature of global MS analysis, a dataset of containing ion amounts with molecular information has great significance when studying a biological system. One popular area that uses proteomics is the development of clinical biomarkers (Jingushi et al., 2017, Nirmalan et al., 2011). Biomarker signatures are defined as an index that can be measured in the body fluids and tissues and used to evaluate objectively the pharmacological responses during normal biological, pathological processes, and practical treatments (Rifai et al., 2006, Vasan, 2006). Moreover, these biomarkers are verified as indicators for the prediction of disease, prognosis, and individual differences. Biomarker rules are roughly classified into three categories: reference limits that statistically derive cut-off points based on the reference samples, discrimination limits that separate patients with a disease from those without it, and threshold follow-ups that identify the level of disease. As a therapeutic index, the blood level monitoring of therapeutic drugs can be visualized, and the treatment responses can be quantified to support practical decisions. Biomarkers can be distinctly subdivided in accordance with their purpose. Starting from broad categories like diagnostic markers or companion diagnosis, biomarkers suitable for a situation have more clinical significance, such as prognostic, predictive, surrogate, monitoring, toxicity, pharmacodynamics (PD), autoantigen, and stratification markers (Poste et al., 2012). The strategies for biomarker discovery differ largely according to the target for the therapeutic or diagnostic phase. A biological system exerts its functions by controlling the expression of many molecules in response to constantly changing phenomena. Even though phenotypic surfaces are silently homeostatic, systems are predicted to be based on various controls in order to maintain the biological process (Kurachi et al., 2009). Taking this situation into consideration, a disease profile and mechanism can be elucidated in detail using an identical biological sample set from the initiation of disease with inflammatory symptoms, pathogenic events to regenerative recovery. Integrated proteomic strategies with protein expression and time-dependent profile changes leads to the discovery of more in-depth biomarkers (Shimada et al., 2010). Quantitative proteomic views and approaches are indispensable for analyzing these systems biology.In this review, we discuss the principles and applications of MS in the context of the trend of quantitative proteomics based on biomolecular-specific structures, and the analytical advances in MS and their contributions to the life science, medical, and pharmaceutical fields. We also describe our recent advances in MS-based bioanalysis and how they have contributed to the remarkable development of antibody drugs and their therapeutic applications.2. Structure-indicated quantitation by mass spectrometry2.1. MS/MS fragmentation, accuracy, and resolution for peptide identificationCurrently, in the proteomic field with MS, accurate protein identification is generally performed using a dataset of precursor and product ions generated by collision-induced dissociation (CID) in hybrid MS function. Peptide fragmentation patterns depend on several factors such as amino acid sequence, covalent potential of amide or other covalent bonds, and ion valence. In a peptide bond dissociation reaction, product ions derived from amide bonds are preferentially cleaved and detected because of the larger dissociation constant of the amide compared to that of residues to α‑carbon, and high probability of charge localization on the amide by weak conjugation formation. Consequently, the detection probability derived from the b- and y-ion series increases. These ion series are most commonly used for structure assignment because they are reflected in peptide-specific amino acid sequences (Guthals & Bandeira, 2012).The atomic content of peptides includes a naturally constant ratio of stable isotopes. For the exact mass, the lowest m/z peak in no isotope distribution, the monoisotopic mass, is selected (Valkenborg, Jansen, & Burzykowski, 2008). The selection of the monoisotopic peak should be carried out as accurately as possible. Peak processing errors lead to a mass difference of 1 Da or more. Proteomic analysis involves a huge number of peptides as analytical targets, and several precursor ions are frequently detected in the near m/z. Therefore, peak processing errors definitely prevent protein identification (DeFelice et al., 2017). On the other hand, high-resolution MS greatly aids in identification and structure assignment. Isoleucine and leucine are isobaric and cannot be separated, but it is possible to distinguish between glutamate and leucine (mass δ = 0.036 Da). Moreover, neutral loss ions of deamidation (δ = 17 Da) and dehydration (δ = 18 Da) can be also reliably separated. As proteomic strategies are designed to be more accurate, high-resolution MS becomes more essential.2.2. Protein identification by mass spectrometry data processingProteomic analysis is assisted seamlessly by excellent MS data processing algorithms. The exact monoisotopic peaks of peptide ions from the protein digestion procedure are acquired, and a peak list of the combination of the precursor and generated product ion information with ion valence is produced. Then, this peak list is entered into the database analysis server, which returns with the ion scores of each peptide. The ion scores of the peptides are calculated using an appropriate scoring algorithm with a theoretical peptide fragment dataset generated from submitted sequence databases. The closest match or matches for a peptide can be identified using the identification criteria of the reliability scores. Currently, Mascot (Matrix Science, UK) (Eng et al., 1994, Pappin et al., 1993), ProteinProspector (UCSF, US) (Chalkley et al., 2005), ProteinPilot (Sciex, US), Proteome Discoverer (Thermo Fisher, US), ProteinScape (Bruker, DE), and Expressionist (Genedata, Switzerland) (Ueda et al., 2011) are the main active well-known proteome servers, and are indispensable engines for large-scale proteomics. For the functional annotation and statistical analysis of identified proteins, Gene Ontology (Gene Ontology Resource) (Camon et al., 2004, Zeeberg et al., 2003) and DABID Bioinformatics (http://david.ncifcrf.gov/) (Huang da, Sherman, & Lempicki, 2009) provide web-accessible engines to visualize biological investigation from several omics dataset. Furthermore, ProteinAtras (http://www.proteinatlas.org/) (Uhlen et al., 2010, Uhlen et al., 2015) and ExSPASy (https://www.expasy.org/) (Artimo et al., 2012) were built as unified protein knowledgebases.The process of data analysis and annotation of large scale dataset from MS is extremely significant for the proteomics-based sciences, which is why, MS is also referred to as an information science.2.3. Comprehensive quantitation for overall systems biologyA quantitative proteomic dataset with high reproducibility provides valuable biological impacts. Specifically, when elucidating a biological landscape as a system, unified strategies combined with multi-omics technologies are necessary. To quantitate the proteins comprehensively, individual ion peaks derived from identified proteins are determined. For more effective and successive quantitation, a targeted quantitation method is currently carried out. The hybrid triple quadrupole (TQ) MS function of selecting a precursor ion from tryptic peptides by the 1st quadrupole (Q1), CID reaction with Argon or a rare gas in the 2nd quadrupole (Q2), and selection of a structure-specific product ion by the 3rd quadrupole (Q3) is optimal for the accurate and successive quantitation of the proteome. The combination of the precursor (Q1) and product ion (Q3) m/z is named the transition of multiple reaction monitoring (MRM) or selected reaction monitoring (SRM). Global quantitative analysis becomes possible by switching this transition at high speed. However, the transition switching speed has an upper limit, and there are some cases in which the MRM method alone cannot perform a satisfactory quantitation of large-scale proteomics due to the prevalence of high-performance LC. Additionally, in recent years, the MRM method has been improved to the parallel reaction monitoring (PRM) (Kreimer et al., 2017). In PRM, a specific product ion is not selected by Q3, but all fragment ions are detected and quantitated after data acquisition. Compared with MRM, the features of PRM encompass a wider range of conditions for quantitative use. Moreover, PRM can be used to clarify the quantitative structure indexes and transition interference from a biological matrix. The database resources PeptideAtras (PeptideAtlas) (Desiere et al., 2006), Skyline (http://skyline.ms/project/home/software/Skyline/begin.view) (MacLean et al., 2010), MaxQuant (http://www.biochem.mpg.de/5111795/maxquant) (Cox & Mann, 2008), and iMPAQT (http://www.bioreg.kyushu-u.ac.jp/saibou/index_en.html) (Matsumoto et al., 2017) are currently being advanced and contributed to the accurate quantitative proteomics.Matsumoto M and Nakayama K et al. have performed excellent study to elucidate the landscape of metabolic systems by identifying and quantifying the global metabolic enzymes on the pathway under the assumption that the expression levels of proteins are the rate-limiting factors in metabolic regulation (Matsumoto et al., 2017). In order to quantitate an individual protein within the genome-wide proteome, they first developed the proteotypic peptide (PTP) (Michalski et al., 2011, Razavi et al., 2012, Selevsek et al., 2015), which can be used to perform the specific quantitation of an individual protein. PTPs are selected from among the tryptic peptides of recombinant human proteins from full-length human cDNA libraries (Goshima et al., 2008). By using actual MS data for the selection of PTPs, optimal PTP selection has become possible through the overall consideration of the efficiency of digestion, peptide release, and ionization. Moreover, the copy number of expressed protein can be determined using the stable isotope-labeled (SIL) PTP as an internal standard (Howden et al., 2013, Ong et al., 2002). Using this strategy, the quantitation of a low-abundance proteome is possible with a wide dynamic range.3. Bioanalytical technologies for protein biopharmaceuticals3.1. Recent advancements in antibody drugs and the necessity of clinical indicators for drug efficacySince the 1980s, specific molecules and pathways related to individual diseases have been identified, and the research to elucidate the molecular mechanism has advanced dramatically. These achievements accelerated the development of molecular target drugs that inhibit a specific molecule and signaling pathway. The approval of anti-CD20 Rituximab for lymphoma (Maloney et al., 1997), anti-ErbB2 Trastuzumab for breast cancer (Baselga, Norton, Albanell, Kim, & Mendelsohn, 1998), and anti-TNFα Infliximab for rheumatoid arthritis (Targan et al., 1997) contributed to the acceleration of the development of antibody drugs in the late 1990s. Recently, antibody drugs against CD antigens, receptors, growth factors, cytokines, immune checkpoints, infectious factors, and amyloid beta have been developed, and about more 60 drugs have been approved as molecular targeted antibody drugs (Mullard, 2017a, Mullard, 2017b). Furthermore, more than 500 items and their biosimilars have been developed, and further improvements are expected. In fact, the combined administration of low-molecular-weight and antibody drugs against a specific disease has become a standard treatment (Avallone et al., 2016). On the other hand, drug efficacy, toxicity, and side effects are correlated with individual differences (Ito, Kondo, Tada, & Kitano, 2015). Thus, treatment has evolved into individualized and optimized medicine in which a patient group that is expected to receive medicinal treatment is selected scientifically before the practical treatment. In the realization of individualized medicine, additional information such as on the expression level of the target molecules, genetic background, indicators of drug efficacy, toxicity biomarkers, immunoreactivity, and metabolism are extremely important.The drug efficacy of small-molecular target drugs typically has a narrow therapeutic window, and blood concentration is one of a key surrogate efficacy index for the appropriate dosage for an individual. Currently, for the anti-cancer agents methotrexate (Przybylski, Preiss, Dennebaum, & Fischer, 1982) and imatinib (Beumer, 2013, Hamada et al., 2003), and pharmaceuticals such as immunosuppressive agents cyclosporine and tacrolimus, it is possible to perform effective treatment maintenance by carrying out therapeutic drug monitoring (Wong, 2001). On the other hand, the metabolic pathway and drug efficacy index of antibody drugs remain unclear, and as a result, the dosage level is not determined enough. However, a positive correlation between the blood concentration and survival was reported about in recent clinical trials of Trastuzumab (Kang et al., 2014) and Ramucirumab (Tabernero et al., 2017) for progressive gastric cancer. Moreover, in a clinical study of Infliximab and Adalimumab against rheumatoid arthritis (Mulleman et al., 2009, Pouw et al., 2015), the blood concentration and inflammation level were inversely correlated. Furthermore, the overproduction of anti-drug antibodies as one of the causes has been addressed (G. P. Eng et al., 2015, Marinari et al., 2014). In 2013, cancer immunotherapy was selected as the breakthrough of the year (Couzin-Frankel, 2013, Ishida et al., 1992): by inhibiting the binding of PD-1/PD-L1, it activates cytotoxic T cells, and lead to the attack to cancer cells that originally escaped from detection by immune system. The antibody drug will most likely be applied to many cancers as the notable treatments of the fourth option, immunotherapy, following surgery, chemotherapy, and radiation. In addition, for the novel therapeutic strategy of controlling the delicate balance of the immune system, the phenotypic response, which had not been anticipated, has been confirmed, and the development of an index that can be used to accurately evaluate efficacy, toxicity, and other factors, has become an urgent issue (Davies and Duffield, 2017, Gao et al., 2012). In the near future, the development of therapeutic methods incorporating scientific indicators for treatment using antibody drugs is required.3.2. Monitoring of protein biopharmaceuticals using current proteomic approachesThe ligand binding assay (LBA) is generally used as a bioanalytical method for monitoring antibody drugs. The LBA method, consists of a specific antibody against an antigen, the purification and concentration of the antigen from the biological matrix, and then a sandwich immunoassay using the detected antibody. Although this detection method mainly involves colorimetric and chemiluminescence produced by an enzymatic reaction such as horseradish peroxidase, an analysis with higher selectivity is possible using MS. This method is called hybrid LBA/LC-MS or the mass spectrometric immunoassay (Trenchevska, Nelson, & Nedelkov, 2016).In bioanalysis using hybrid LBA/LC-MS, in addition to the evaluation of selectivity and the recovery rate from the matrix used in the usual validation test, it is also necessary to validate the affinity capture efficiency of the enzyme and the digestion efficiency. Moreover, in the LBA, it is very important to assess the lot-to-lot variability of the capturing antibody or ligand. In consideration of these points, to establish the internal standard material, the SIL of authentic samples of the full length of the antibody to be analyzed is an ideal procedure (Ismaiel, Mylott, & Jenkins, 2017). Furthermore, the accelerated production of endogenous antibodies against antibody drugs is considered to interfere with precise measurements (Bader et al., 2017). In particular, it is possible to directly affect the affinity capture efficiency. To address this issue, the whole digest samples from the serum are used, and the signature peptide to be selected from the LC-MS is quantified based on the technology of conventional shotgun proteomics. With this technique, it is possible to perform a bioanalysis dependent only on the selectivity potential of high performance MS.3.3. Novel approach of Fab-selective proteolysis nSMOLThe method of LBA or hybrid LBA/LC-MS requires an affinity antibody against each antibody drug and it has become difficult to maintain the analytical the quality in the recent clinical advancement. We confirmed that the influence on the MS quantified value and its behavior differ depending on the type of idiotypic antibody against each antibody drug (Iwamoto, Hamada, & Shimada, 2017). This suggests that it is difficult to prepare an appropriate affinity antibody clones or specific ligands for hybrid LBA/LC-MS, and confirmation of the actual value is essential for the individual clinical difference. And by the whole digest approaches, it is difficult to maintain the ESI interface clearance because complex biological samples have a large excess of peptide analytes (Liu et al., 2012). In the protein bioanalysis using MS, there are several principle issues to overcome, such as the problem of separation ability and carryover in the chromatograph portion, the ion suppression effect and desolvation efficiency of the target molecule due to coexisting ions in the MS, and the handling of multiple sample processing. Accordingly, the development of bioanalytical methods, which make use of the features of MS such as high selectivity, completeness, and continuity, is needed involving the overall optimization of sample processing, biochemistry, structure, enzyme reaction and release efficiency, material sciences, column separation, ionization efficiency, and throughput ability, to contribute to clinical chemistry and pharmaceutical science.We proposed a novel analytical method based on the structure specificity of an antibody independent of a variety of monoclonal antibodies, and this basic technology has been widely applicable to the development and clinical use of antibody drugs. Immunoglobulin G (IgG) is the main antibody drug that has been analyzed. IgG has a 15 nm molecular diameter and is tetramer protein that has two heavy and light chains. IgG is the second major proteome in blood protein content. Since the structural features representing the antigen specificity of antibodies are determined by amino acid substitution through somatic hypermutation on the variable fragment Fv (Novotny and Bruccoleri, 1987, Wu and Kabat, 1970), universal antibody analysis becomes possible by selectively quantitating the complementarity-determining regions (CDRs). Based on these considerations, we developed a novel method, nano-surface and molecular-orientation limited (nSMOL) proteolysis (Iwamoto et al., 2014), for the bioanalysis of antibody drugs (Fig. 2). The nSMOL method consists of orientating the fragment antigen-binding (Fab) towards the reaction solution side by binding IgG in a porous body with a pore diameter of 100 nm via the constant region Fc, and immobilizing the protease on the surface of the nanoparticles with a diameter of 200 nm, resulting in physicochemically limiting the access of the protease to the immobilizing IgG substrate. In this solid-solid reaction, Fab-selective proteolysis becomes possible, and consequently, it is possible to greatly reduce the complexity of the peptide to be analyzed by holding the structural specificity of IgG and eliminating the contamination of the protease. The peptides that include CDRs are candidate signature peptides for antibody drug determination and quantitation, and it is possible to provide high sensitivity, high selectivity, and universal use of analytical technology with appropriately selecting MRM transitions that have antibody structure specificity.Fig. 2 .Fig. 2. Concept representation of nSMOL proteolysis.IgG molecules are collected in the porous resin with 100 nm diameter via fragment, crystallizable (Fc), so that fragment, antigen-binding (Fab) is oriented towards reaction solution. Modified trypsin (shown in yellow picture) is immobilized on the surface of nanoparticle with 200 nm diameter. In this solid-solid reaction, trypsin access to IgG substrate is physically limited, and Fab-selective proteolysis successfully continues. Consequently, it is possible to greatly reduce the complexity of the peptide analyte by holding the structural specificity of IgG on CDR and eliminating contamination of protease, and providing the high-sensitivity and high-selectivity quantitation of universal antibody bioanalysis. (For interpretation of the references to colour in this figure legend, the reader is referred to the web version of this article.)Even the good antibody with high-specificity and high-affinity to the ligand, bioanalytical validation using the common LBA-related methods surely require the one antibody substrate for one ligand. This one-to-one approach is not only difficult to apply into the broad and rapid clinical studies, but also the cost for preparation and quality control of biological components become a critical issue. However, using Fab-selective reaction nSMOL coupled with mass-based resolution LC-MS/MS, bioanalytical validation for many monoclonal antibodies become possible on the identical platform. And the nSMOL can be configured the condition setting independent of animal matrices. This feature means that comparative data analysis may be addressed from the initial stage of antibody development in animal model to the first-in-human and late-phase clinical trials, and the contribution to antibody drug discovery. Moreover, this strategy can accelerate the cross-sectional trials on rare diseases, and drug-drug interaction assessment for the new combination therapy.The analytical reliability of antibody drug analysis using nSMOL is based on the “Enforcement Regulations of the Law Concerning the Quality, Effectiveness and Security of Securing Drugs, Medical Equipment, etc.” and “Guideline on Bioanalytical Method Validation in Pharmaceutical Development”. In order to verify that nSMOL proteolysis accompanied by TQ-LC-MS analysis meets the criteria of these guideline, the authors have already carried out and reported on the full validation analysis against various antibody drugs (Trastuzumab (Iwamoto, Yamane, Umino, Hamada, & Shimada, 2015), Bevacizumab (Iwamoto, Umino, et al., 2016), Cetuximab (Iwamoto, Takanashi, et al., 2016), Rituximab (Iwamoto, Takanashi, Hamada, & Shimada, 2016b), Nivolumab (Iwamoto, Shimada, Terakado, and Hamada, 2016), Brentuximab vedotin (Iwamoto, Takanashi, Hamada, & Shimada, 2016a), and Trastuzumab emtansine (Iwamoto, Shimomura, Tamura, Hamada, & Shimada, 2017)) using nSMOL. Moreover, since the nSMOL reacts to whole IgG molecules in the blood in principle, multiplex quantitation is possible for the application of combination cancer therapy and therapeutic drug monitoring for inflammatory immune diseases (Iwamoto et al., 2016d, Messenheimer et al., 2017, Steenholdt et al., 2014). nSMOL results have demonstrated that blood concentrations in the range of about 0.1 to 300 μg/mL fulfill all validation criteria for antibody drugs, and it has both analytical reliability and multipurpose universal use (Table 1). This quantifiable range will fulfill without the requirements of any additional operations such as dilution and covers clinical dosage levels and pharmacokinetic (PK) studies, which can be expected to be widely applicable to antibody drug monitoring.Table 1. Fully validated antibodies by nSMOL coupled with LC-MS/MS bioanalysis. Quantitative information in human plasma for individual signature peptide sequence, MRM transition, and linear dynamic range are summarized.Antibodiesa Signature peptide sequences MRM transition Quantitation range in plasma[μg/mL]Precursor ionQ1 [m/z] Product ionQ3 [m/z]Trastuzumab IYPTNGYTR 542.8 404.7 0.06–250Bevacizumab FTFSLDTSK 523.3 797.4 0.15–300Cetuximab SQVFFK 378.2 540.3 0.58–300Rituximab ASGYTFTSYNMHWVK 598.1 817.5 0.58–300Nivolumab ASGITFSNSGMHWVR 550.8 661.5 0.15–250Brentuximab vedotin VLIYAASNLESGIPAR 837.5 343.1 0.58–300Trastuzumab emtansine IYPTNGYTR 542.8 404.7 0.06–250aDetail bioanalytical conditions for each antibody are described in: Trastuzumab (Iwamoto et al., 2015); Bevacizumab (Iwamoto, Umino, et al., 2016); Cetuximab (Iwamoto, Takanashi, et al., 2016); Rituximab (Iwamoto et al., 2016b); Nivolumab (Iwamoto, Shimada, et al., 2016); Brentuximab vedotin (Iwamoto et al., 2016a); Trastuzumab emtansine (Iwamoto et al., 2017).4. Summary and future aspectsThe analytical data obtained from MS include the structure-derived ions of measured molecules, but it can be developed into a wide variety of research applications by correctly understanding and applying the MS data. Proteomics, which is a necessary MS technology, is an optimal strategy for understanding the complicated systems biology (Bakalarski & Kirkpatrick, 2016). Although a part of the actual approach has not been generally used and applied, proteomics has become a cutting-edge scientific field, which opens new avenues for life sciences on the premise of coverage, by combining various aspects of biochemistry, analytical chemistry, physical chemistry, material science, and informatics with the structure specificity of biomolecules as an indicator. Specifically, in addition to not only structural information and molecular identification information, but also quantitative information can be obtained, which makes it possible to integrate with superior database, and it has led to great progress in understanding biology. The accumulation of quantitative proteome analysis technology is informative, and due to improvements in liquid chromatography technologies, the range of applications has dramatically expanded. The antibody drug monitoring technology nSMOL, which we recently developed, is also a technology conceived as a result of addressing the fundamental technologies of proteomics and clinical and pharmaceutical issues.Although one medicinal efficacy indicators for antibody drugs, blood concentration, is important, it is not enough. We consider that the technologies related to the estimation of lesion distribution, quantification of anti-drug antibodies and search for metabolic pathways, have the potential to open doors in research fields on the PK of antibody drugs, for which there remain many unknowns.In the future, the development of new indicators and surrogate markers is required to advance to personalized medicine and the dosing strategy of highly efficient pharmaceuticals. For the development of indicators in new fields, such as the quantification of major histocompatibility complex (MHC) peptide complex, the high efficiency identification of short chain peptides, development of exosome markers, immune cell monitoring, MS technology, and improvement of ionization reaction efficiency, will become significant areas (Caron et al., 2015, Chen and Mellman, 2013). We hope that the analytical technology of MS will expand in parallel with these advances.Conflict of interest statementAll the authors are employees of SHIMADZU Corporation, and declare no conflicts of interest associated with this manuscript.AcknowledgmentsThis review includes a part of results from research collaborations. The authors sincerely appreciate the valuable discussions with Dr. Koichi Tanaka of SHIMADZU Corporation, Dr. Masaya Ikegawa of Doshisha University, Dr. Akinobu Hamada and Dr. Hitoshi Nakagama of the National Cancer Center, and Dr. Atsushi Yonezawa of Kyoto University.ReferencesAebersold and Mann, 2016R. Aebersold, M. MannMass-spectrometric exploration of proteome structure and functionNature, 537 (2016), pp. 347-355CrossRefView Record in ScopusGoogle ScholarAnderson and Anderson, 2002N.L. Anderson, N.G. AndersonThe human plasma proteome: History, character, and diagnostic prospectsMolecular & Cellular Proteomics, 1 (2002), pp. 845-867View Record in ScopusGoogle ScholarArtimo et al., 2012P. Artimo, M. Jonnalagedda, K. Arnold, D. Baratin, G. Csardi, E. de Castro, ..., H. StockingerExPASy: SIB bioinformatics resource portalNucleic Acids Research, 40 (2012), pp. W597-603CrossRefView Record in ScopusGoogle ScholarAvallone et al., 2016A. Avallone, M.C. Piccirillo, L. Aloj, G. Nasti, P. Delrio, F. Izzo, ..., A. BudillonA randomized phase 3 study on the optimization of the combination of bevacizumab with FOLFOX/OXXEL in the treatment of patients with metastatic colorectal cancer—OBELICS (Optimization of BEvacizumab scheduLIng within Chemotherapy Scheme)BMC Cancer, 16 (2016), p. 69Google ScholarBader et al., 2017L.I. Bader, S.M. Solberg, S.H. Kaada, N. Bolstad, D.J. Warren, S. Gavasso, ..., C.A. VedelerAssays for infliximab drug levels and antibodies: A matter of scales and categoriesScandinavian Journal of Immunology, 86 (2017), pp. 165-170CrossRefView Record in ScopusGoogle ScholarBakalarski and Kirkpatrick, 2016C.E. Bakalarski, D.S. KirkpatrickA biologist's field guide to multiplexed quantitative proteomicsMolecular & Cellular Proteomics, 15 (2016), pp. 1489-1497View Record in ScopusGoogle ScholarBaselga et al., 1998J. Baselga, L. Norton, J. Albanell, Y.M. Kim, J. MendelsohnRecombinant humanized anti-HER2 antibody (Herceptin) enhances the antitumor activity of paclitaxel and doxorubicin against HER2/neu overexpressing human breast cancer xenograftsCancer Research, 58 (1998), pp. 2825-2831View Record in ScopusGoogle ScholarBeumer, 2013J.H. BeumerWithout therapeutic drug monitoring, there is no personalized cancer careClinical Pharmacology and Therapeutics, 93 (2013), pp. 228-230CrossRefView Record in ScopusGoogle ScholarCalderon-Celis et al., 2017F. Calderon-Celis, J.R. Encinar, A. Sanz-MedelStandardization approaches in absolute quantitative proteomics with mass spectrometryMass Spectrometry Reviews (2017), pp. 1-23, 10.1002/mas.21542View Record in ScopusGoogle ScholarCamon et al., 2004E. Camon, M. Magrane, D. Barrell, V. Lee, E. Dimmer, J. Maslen, ..., R. ApweilerThe Gene Ontology Annotation (GOA) database: Sharing knowledge in Uniprot with gene ontologyNucleic Acids Research, 32 (2004), pp. D262-266View Record in ScopusGoogle ScholarCaron et al., 2015E. Caron, D.J. Kowalewski, C. Chiek Koh, T. Sturm, H. Schuster, R. AebersoldAnalysis of Major Histocompatibility Complex (MHC) immunopeptidomes using mass spectrometryMolecular & Cellular Proteomics, 14 (2015), pp. 3105-3117View Record in ScopusGoogle ScholarCaron et al., 2011E. Caron, K. Vincent, M.H. Fortier, J.P. Laverdure, A. Bramoulle, M.P. Hardy, ..., C. PerreaultThe MHC I immunopeptidome conveys to the cell surface an integrative view of cellular regulationMolecular Systems Biology, 7 (2011), p. 533CrossRefGoogle ScholarChalkley et al., 2005R.J. Chalkley, P.R. Baker, L. Huang, K.C. Hansen, N.P. Allen, M. Rexach, A.L. BurlingameComprehensive analysis of a multidimensional liquid chromatography mass spectrometry dataset acquired on a quadrupole selecting, quadrupole collision cell, time-of-flight mass spectrometer: II. New developments in Protein Prospector allow for reliable and comprehensive automatic analysis of large datasetsMolecular & Cellular Proteomics, 4 (2005), pp. 1194-1204CrossRefView Record in ScopusGoogle ScholarChen and Mellman, 2013D.S. Chen, I. MellmanOncology meets immunology: The cancer-immunity cycleImmunity, 39 (2013), pp. 1-10ArticleDownload PDFCrossRefView Record in ScopusGoogle ScholarCouzin-Frankel, 2013J. Couzin-FrankelBreakthrough of the year 2013. Cancer immunotherapyScience, 342 (2013), pp. 1432-1433CrossRefView Record in ScopusGoogle ScholarCox and Mann, 2008J. Cox, M. MannMaxQuant enables high peptide identification rates, individualized p.p.b.-range mass accuracies and proteome-wide protein quantificationNature Biotechnology, 26 (2008), pp. 1367-1372CrossRefView Record in ScopusGoogle ScholarDavies and Duffield, 2017M. Davies, E.A. DuffieldSafety of checkpoint inhibitors for cancer treatment: Strategies for patient monitoring and management of immune-mediated adverse eventsImmunotargets and Therapy, 6 (2017), pp. 51-71CrossRefView Record in ScopusGoogle ScholarDeFelice et al., 2017B.C. DeFelice, S.S. Mehta, S. Samra, T. Cajka, B. Wancewicz, J.F. Fahrmann, O. FiehnMass Spectral Feature List Optimizer (MS-FLO): A tool to minimize false positive peak reports in untargeted Liquid Chromatography-Mass Spectroscopy (LC-MS) data processingAnalytical Chemistry, 89 (2017), pp. 3250-3255CrossRefView Record in ScopusGoogle ScholarDesiere et al., 2006F. Desiere, E.W. Deutsch, N.L. King, A.I. Nesvizhskii, P. Mallick, J. Eng, ..., R. AebersoldThe PeptideAtlas projectNucleic Acids Research, 34 (2006), pp. D655-658View Record in ScopusGoogle ScholarEng et al., 2015G.P. Eng, K. Bendtzen, H. Bliddal, M. Stoltenberg, M. Szkudlarek, V. Fana, ..., P.N. BoucheloucheAntibodies to infliximab and adalimumab in patients with rheumatoid arthritis in clinical remission: A cross-sectional studyArt, 2015 (2015), p. 784825View Record in ScopusGoogle ScholarEng et al., 1994J.K. Eng, A.L. McCormack, J.R. YatesAn approach to correlate tandem mass spectral data of peptides with amino acid sequences in a protein databaseJournal of the American Society for Mass Spectrometry, 5 (1994), pp. 976-989ArticleDownload PDFCrossRefView Record in ScopusGoogle ScholarFrancavilla et al., 2017C. Francavilla, M. Lupia, K. Tsafou, A. Villa, K. Kowalczyk, R. Rakownikow Jersie-Christensen, ..., J.V. OlsenPhosphoproteomics of primary cells reveals druggable kinase signatures in ovarian cancerCell Reports, 18 (2017), pp. 3242-3256ArticleDownload PDFView Record in ScopusGoogle ScholarFukuyama et al., 2008Y. Fukuyama, S. Nakaya, Y. Yamazaki, K. TanakaIonic liquid matrixes optimized for MALDI-MS of sulfated/sialylated/neutral oligosaccharides and glycopeptidesAnalytical Chemistry, 80 (2008), pp. 2171-2179CrossRefView Record in ScopusGoogle ScholarGao et al., 2012B. Gao, S. Yeap, A. Clements, B. Balakrishnar, M. Wong, H. GurneyEvidence for therapeutic drug monitoring of targeted anticancer therapiesJournal of Clinical Oncology, 30 (2012), pp. 4017-4025View Record in ScopusGoogle ScholarGhaste et al., 2016M. Ghaste, R. Mistrik, V. ShulaevApplications of Fourier Transform Ion Cyclotron Resonance (FT-ICR) and orbitrap based high resolution mass spectrometry in metabolomics and lipidomicsInternational Journal of Molecular Sciences, 17 (2016)Google ScholarGiansanti et al., 2016P. Giansanti, L. Tsiatsiani, T.Y. Low, A.J. HeckSix alternative proteases for mass spectrometry-based proteomics beyond trypsinNature Protocols, 11 (2016), pp. 993-1006CrossRefView Record in ScopusGoogle ScholarGoshima et al., 2008N. Goshima, Y. Kawamura, A. Fukumoto, A. Miura, R. Honma, R. Satoh, ..., N. NomuraHuman protein factory for converting the transcriptome into an in vitro-expressed proteomeNature Methods, 5 (2008), pp. 1011-1017CrossRefView Record in ScopusGoogle ScholarGuthals and Bandeira, 2012A. Guthals, N. BandeiraPeptide identification by tandem mass spectrometry with alternate fragmentation modesMolecular & Cellular Proteomics, 11 (2012), pp. 550-557View Record in ScopusGoogle ScholarHamada et al., 2003A. Hamada, H. Miyano, H. Watanabe, H. SaitoInteraction of imatinib mesilate with human P-glycoproteinThe Journal of Pharmacology and Experimental Therapeutics, 307 (2003), pp. 824-828View Record in ScopusGoogle ScholarHardman and Makarov, 2003M. Hardman, A.A. MakarovInterfacing the orbitrap mass analyzer to an electrospray ion sourceAnalytical Chemistry, 75 (2003), pp. 1699-1705View Record in ScopusGoogle ScholarHommerson et al., 2011P. Hommerson, A.M. Khan, G.J. de Jong, G.W. SomsenIonization techniques in capillary electrophoresis-mass spectrometry: Principles, design, and applicationMass Spectrometry Reviews, 30 (2011), pp. 1096-1120CrossRefView Record in ScopusGoogle ScholarHowden et al., 2013A.J. Howden, V. Geoghegan, K. Katsch, G. Efstathiou, B. Bhushan, O. Boutureira, ..., O. AcutoQuaNCAT: Quantitating proteome dynamics in primary cellsNature Methods, 10 (2013), pp. 343-346CrossRefView Record in ScopusGoogle ScholarHowells et al., 2005L.C. Howells, A.O. Whelan, M.J. Woodward, N.G. ColdhamSimple and effective technical procedures for the packing and application of nanospray PicoFrit HPLC columns for proteomic analysesProteomics, 5 (2005), pp. 3864-3867CrossRefView Record in ScopusGoogle ScholarHuang da et al., 2009W. Huang da, B.T. Sherman, R.A. LempickiSystematic and integrative analysis of large gene lists using DAVID bioinformatics resourcesNature Protocols, 4 (2009), pp. 44-57View Record in ScopusGoogle ScholarIshida et al., 1992Y. Ishida, Y. Agata, K. Shibahara, T. HonjoInduced expression of PD-1, a novel member of the immunoglobulin gene superfamily, upon programmed cell deathEMBO Journal, 11 (1992), pp. 3887-3895View Record in ScopusGoogle ScholarIsmaiel et al., 2017O.A. Ismaiel, W.R. Mylott Jr., R.G. JenkinsDo we have a mature LC-MS/MS methodology for therapeutic monoclonal antibody bioanalysis?Bioanalysis, 9 (2017), pp. 1289-1292CrossRefView Record in ScopusGoogle ScholarIto et al., 2015A. Ito, S. Kondo, K. Tada, S. KitanoClinical development of immune checkpoint inhibitorsBioMed Research International, 2015 (2015), p. 605478Google ScholarIwamoto et al., 2017N. Iwamoto, A. Hamada, T. ShimadaAntibody drug quantitation in coexistence with anti-drug antibodies on nSMOL bioanalysisAnalytical Biochemistry, 540-541 (2017), pp. 30-37Google ScholarIwamoto et al., 2016N. Iwamoto, T. Shimada, H. Terakado, A. HamadaValidated LC-MS/MS analysis of immune checkpoint inhibitor Nivolumab in human plasma using a Fab peptide-selective quantitation method: Nano-surface and molecular-orientation limited (nSMOL) proteolysisJournal of Chromatography. B, Analytical Technologies in the Biomedical and Life Sciences, 1023-1024 (2016), pp. 9-16ArticleDownload PDFView Record in ScopusGoogle ScholarIwamoto et al., 2014N. Iwamoto, T. Shimada, Y. Umino, C. Aoki, Y. Aoki, T.A. Sato, ..., H. NakagamaSelective detection of complementarity-determining regions of monoclonal antibody by limiting protease access to the substrate: Nano-surface and molecular-orientation limited proteolysisAnalyst, 139 (2014), pp. 576-580CrossRefGoogle ScholarIwamoto et al., 2017N. Iwamoto, A. Shimomura, K. Tamura, A. Hamada, T. ShimadaLC-MS bioanalysis of Trastuzumab and released emtansine using nano-surface and molecular-orientation limited (nSMOL) proteolysis and liquid-liquid partition in plasma of Trastuzumab emtansine-treated breast cancer patientsJournal of Pharmaceutical and Biomedical Analysis, 145 (2017), pp. 33-39ArticleDownload PDFView Record in ScopusGoogle ScholarIwamoto et al., 2016dN. Iwamoto, M. Takanashi, A. Hamada, T. ShimadaMultiplex LCMS bioanalysis of Brentuximab Vedotin, Rituximab and Cetuximab towards therapeutic drug monitoring application by combined calibration curve using Fab-selective limited proteolysis nSMOLClinical Pharmacology & Biopharmaceutics, 5 (2016)Google ScholarIwamoto et al., 2016eN. Iwamoto, M. Takanashi, A. Hamada, T. ShimadaValidated LC/MS bioanalysis of Rituximab CDR peptides using nano-surface and Molecular-Orientation Limited (nSMOL) proteolysisBiological & Pharmaceutical Bulletin, 39 (2016), pp. 1187-1194CrossRefView Record in ScopusGoogle ScholarIwamoto et al., 2016N. Iwamoto, M. Takanashi, Y. Umino, C. Aoki, A. Hamada, T. ShimadaApplication of nano-surface and molecular-orientation limited proteolysis to LC-MS bioanalysis of cetuximabBioanalysis, 8 (2016), pp. 1009-1020CrossRefView Record in ScopusGoogle ScholarIwamoto et al., 2016N. Iwamoto, Y. Umino, C. Aoki, N. Yamane, A. Hamada, T. ShimadaFully validated LCMS bioanalysis of Bevacizumab in human plasma using nano-surface and molecular-orientation limited (nSMOL) proteolysisDrug Metabolism and Pharmacokinetics, 31 (2016), pp. 46-50ArticleDownload PDFView Record in ScopusGoogle ScholarIwamoto et al., 2015N. Iwamoto, N. Yamane, Y. Umino, A. Hamada, T. ShimadaDevelopment of the validated LCMS bioanalysis of Trastuzumab in human plasma using selective detection method for complementarity-determining regions of monoclonal antibody: Nano-surface and molecular-orientation limited (nSMOL) proteolysisAnalytical Methods, 7 (2015), pp. 9177-9183CrossRefView Record in ScopusGoogle ScholarIwasaki et al., 2010M. Iwasaki, S. Miwa, T. Ikegami, M. Tomita, N. Tanaka, Y. IshihamaOne-dimensional capillary liquid chromatographic separation coupled with tandem mass spectrometry unveils the Escherichia coli proteome on a microarray scaleAnalytical Chemistry, 82 (2010), pp. 2616-2620CrossRefView Record in ScopusGoogle ScholarJingushi et al., 2017K. Jingushi, M. Uemura, N. Ohnishi, W. Nakata, K. Fujita, T. Naito, ..., K. UedaExtracellular vesicles isolated from human renal cell carcinoma tissues disrupt vascular endothelial cell morphology via azurocidinInternational Journal of Cancer, 142 (3) (2017), pp. 607-617Google ScholarKang et al., 2014Y.K. Kang, S.Y. Rha, P. Tassone, J. Barriuso, R. Yu, T. Szado, ..., Y.J. BangA phase IIa dose-finding and safety study of first-line pertuzumab in combination with trastuzumab, capecitabine and cisplatin in patients with HER2-positive advanced gastric cancerBritish Journal of Cancer, 111 (2014), pp. 660-666CrossRefView Record in ScopusGoogle ScholarKirley et al., 2016T.L. Kirley, K.D. Greis, A.B. NormanStructural characterization of expressed monoclonal antibodies by single sample mass spectral analysis after IdeS proteolysisBiochemical and Biophysical Research Communications, 477 (2016), pp. 363-368ArticleDownload PDFView Record in ScopusGoogle ScholarKreimer et al., 2017S. Kreimer, Y. Gao, S. Ray, M. Jin, Z. Tan, N.A. Mussa, ..., B.L. KargerHost cell protein profiling by targeted and untargeted analysis of data independent acquisition mass spectrometry data with parallel reaction monitoring verificationAnalytical Chemistry, 89 (2017), pp. 5294-5302CrossRefView Record in ScopusGoogle ScholarKurachi et al., 2009S. Kurachi, J.S. Huo, A. Ameri, K. Zhang, A.C. Yoshizawa, K. KurachiAn age-related homeostasis mechanism is essential for spontaneous amelioration of hemophilia B LeydenProceedings of the National Academy of Sciences of the United States of America, 106 (2009), pp. 7921-7926CrossRefView Record in ScopusGoogle ScholarLam et al., 2007T.C. Lam, K.K. Li, S.C. Lo, J.A. Guggenheim, C.H. ToApplication of fluorescence difference gel electrophoresis technology in searching for protein biomarkers in chick myopiaJournal of Proteome Research, 6 (2007), pp. 4135-4149CrossRefView Record in ScopusGoogle ScholarLapek Jr. et al., 2017J.D. Lapek Jr., P. Greninger, R. Morris, A. Amzallag, I. Pruteanu-Malinici, C.H. Benes, W. HaasDetection of dysregulated protein-association networks by high-throughput proteomics predicts cancer vulnerabilitiesNature Biotechnology, 35 (2017), pp. 983-989CrossRefView Record in ScopusGoogle ScholarLiu et al., 2012Y. Liu, Z. Miao, R. Lakshmanan, R.R. Ogorzalek Loo, J.A. Loo, H. ChenSignal and charge enhancement for protein analysis by liquid chromatography-mass spectrometry with desorption electrospray ionizationInternational Journal of Mass Spectrometry, 325-327 (2012), pp. 161-166ArticleDownload PDFView Record in ScopusGoogle ScholarMacLean et al., 2010B. MacLean, D.M. Tomazela, N. Shulman, M. Chambers, G.L. Finney, B. Frewen, ..., M.J. MacCossSkyline: An open source document editor for creating and analyzing targeted proteomics experimentsBioinformatics, 26 (2010), pp. 966-968CrossRefView Record in ScopusGoogle ScholarMaloney et al., 1997D.G. Maloney, A.J. Grillo-Lopez, C.A. White, D. Bodkin, R.J. Schilder, J.A. Neidhart, ..., R. LevyIDEC-C2B8 (Rituximab) anti-CD20 monoclonal antibody therapy in patients with relapsed low-grade non-Hodgkin's lymphomaBlood, 90 (1997), pp. 2188-2195View Record in ScopusGoogle ScholarMarinari et al., 2014B. Marinari, E. Botti, M. Bavetta, G. Spallone, A. Zangrilli, M. Talamonti, ..., A. CostanzoDetection of adalimumab and anti-adalimumab levels by ELISA: Clinical considerationsDrug Development Research, 75 (Suppl. 1) (2014), pp. S11-14CrossRefView Record in ScopusGoogle ScholarMartin et al., 2007F.P. Martin, M.E. Dumas, Y. Wang, C. Legido-Quigley, I.K. Yap, H. Tang, ..., J.K. NicholsonA top-down systems biology view of microbiome-mammalian metabolic interactions in a mouse modelMolecular Systems Biology, 3 (2007), p. 112CrossRefGoogle ScholarMatsumoto et al., 2017M. Matsumoto, F. Matsuzaki, K. Oshikawa, N. Goshima, M. Mori, Y. Kawamura, ..., K.I. NakayamaA large-scale targeted proteomics assay resource based on an in vitro human proteomeNature Methods, 14 (2017), pp. 251-258CrossRefView Record in ScopusGoogle ScholarMessenheimer et al., 2017D.J. Messenheimer, S.M. Jensen, M.E. Afentoulis, K.W. Wegmann, Z. Feng, D.J. Friedman, ..., B.A. FoxTiming of PD-1 blockade is critical to effective combination immunotherapy with anti-OX40Clinical Cancer Research, 23 (2017), pp. 6165-6177View Record in ScopusGoogle ScholarMichalski et al., 2011A. Michalski, J. Cox, M. MannMore than 100,000 detectable peptide species elute in single shotgun proteomics runs but the majority is inaccessible to data-dependent LC-MS/MSJournal of Proteome Research, 10 (2011), pp. 1785-1793CrossRefView Record in ScopusGoogle ScholarMora et al., 2000J.F. Mora, G.J. Van Berkel, C.G. Enke, R.B. Cole, M. Martinez-Sanchez, J.B. FennElectrochemical processes in electrospray ionization mass spectrometryJournal of Mass Spectrometry, 35 (2000), pp. 939-952CrossRefGoogle ScholarMorris et al., 1982H.R. Morris, M. Panico, A. Karplus, P.E. Lloyd, B. RinikerElucidation by FAB-MS of the structure of a new cardioactive peptide from AplysiaNature, 300 (1982), pp. 643-645CrossRefView Record in ScopusGoogle ScholarMullard, 2017aA. Mullard2016 FDA drug approvalsNature Reviews. Drug Discovery, 16 (2017), pp. 73-76CrossRefView Record in ScopusGoogle ScholarMullard, 2017bA. MullardBispecific antibody pipeline moves beyond oncologyNature Reviews. Drug Discovery, 16 (11) (2017), p. 810CrossRefGoogle ScholarMulleman et al., 2009D. Mulleman, J.C. Meric, G. Paintaud, E. Ducourau, C. Magdelaine-Beuzelin, J.P. Valat, ..., Centre National de la Recherche Scientifique, U. GInfliximab concentration monitoring improves the control of disease activity in rheumatoid arthritisArthritis Research & Therapy, 11 (2009), p. R178CrossRefGoogle ScholarNirmalan et al., 2011N.J. Nirmalan, C. Hughes, J. Peng, T. McKenna, J. Langridge, D.A. Cairns, ..., R.E. BanksInitial development and validation of a novel extraction method for quantitative mining of the formalin-fixed, paraffin-embedded tissue proteome for biomarker investigationsJournal of Proteome Research, 10 (2011), pp. 896-906CrossRefGoogle ScholarNovotny and Bruccoleri, 1987J. Novotny, R.E. BruccoleriCorrelation among sites of limited proteolysis, enzyme accessibility and segmental mobilityFEBS Letters, 211 (1987), pp. 185-189ArticleDownload PDFCrossRefView Record in ScopusGoogle ScholarOka et al., 1991H. Oka, Y. Ikai, N. Kawamura, J. Hayakawa, K. Harada, H. Murata, ..., Y. ItoDirect interfacing of high-speed countercurrent chromatography to frit electron ionization, chemical ionization, and fast atom bombardment mass spectrometryAnalytical Chemistry, 63 (1991), pp. 2861-2865CrossRefView Record in ScopusGoogle ScholarOng et al., 2002S.E. Ong, B. Blagoev, I. Kratchmarova, D.B. Kristensen, H. Steen, A. Pandey, M. MannStable isotope labeling by amino acids in cell culture, SILAC, as a simple and accurate approach to expression proteomicsMolecular & Cellular Proteomics, 1 (2002), pp. 376-386CrossRefView Record in ScopusGoogle ScholarPappin et al., 1993D.J. Pappin, P. Hojrup, A.J. BleasbyRapid identification of proteins by peptide-mass fingerprintingCurrent Biology, 3 (1993), pp. 327-332ArticleDownload PDFView Record in ScopusGoogle ScholarPoste et al., 2012G. Poste, D.P. Carbone, D.R. Parkinson, J. Verweij, S.M. Hewitt, J.M. JessupLeveling the playing field: Bringing development of biomarkers and molecular diagnostics up to the standards for drug developmentClinical Cancer Research, 18 (2012), pp. 1515-1523CrossRefView Record in ScopusGoogle ScholarPouw et al., 2015M.F. Pouw, C.L. Krieckaert, M.T. Nurmohamed, D. van der Kleij, L. Aarden, T. Rispens, G. WolbinkKey findings towards optimising adalimumab treatment: The concentration-effect curveAnnals of the Rheumatic Diseases, 74 (2015), pp. 513-518CrossRefView Record in ScopusGoogle ScholarPrzybylski et al., 1982M. Przybylski, J. Preiss, R. Dennebaum, J. FischerIdentification and quantitation of methotrexate and methotrexate metabolites in clinical high-dose therapy by high pressure liquid chromatography and field desorption mass spectrometryBiomedical Mass Spectrometry, 9 (1982), pp. 22-32CrossRefView Record in ScopusGoogle ScholarRamani et al., 2008A.K. Ramani, Z. Li, G.T. Hart, M.W. Carlson, D.R. Boutz, E.M. MarcotteA map of human protein interactions derived from co-expression of human mRNAs and their orthologsMolecular Systems Biology, 4 (2008), p. 180CrossRefGoogle ScholarRazavi et al., 2012M. Razavi, L.E. Frick, W.A. LaMarr, M.E. Pope, C.A. Miller, N.L. Anderson, T.W. PearsonHigh-throughput SISCAPA quantitation of peptides from human plasma digests by ultrafast, liquid chromatography-free mass spectrometryJournal of Proteome Research, 11 (2012), pp. 5642-5649CrossRefView Record in ScopusGoogle ScholarRifai et al., 2006N. Rifai, M.A. Gillette, S.A. CarrProtein biomarker discovery and validation: The long and uncertain path to clinical utilityNature Biotechnology, 24 (2006), pp. 971-983CrossRefView Record in ScopusGoogle ScholarSelevsek et al., 2015N. Selevsek, C.Y. Chang, L.C. Gillet, P. Navarro, O.M. Bernhardt, L. Reiter, ..., R. AebersoldReproducible and consistent quantification of the Saccharomyces cerevisiae proteome by SWATH-mass spectrometryMolecular & Cellular Proteomics, 14 (2015), pp. 739-749View Record in ScopusGoogle ScholarShimada et al., 2010T. Shimada, T. Nakanishi, A. Toyama, S. Yamauchi, A. Kanzaki, H. Fujiwake, ..., M. IkegawaPotential implications for monitoring serum bile acid profiles in circulation with serum proteome for carbon tetrachloride-induced liver injury/regeneration model in miceJournal of Proteome Research, 9 (2010), pp. 4490-4500CrossRefView Record in ScopusGoogle ScholarStanding, 2003K.G. StandingPeptide and protein de novo sequencing by mass spectrometryCurrent Opinion in Structural Biology, 13 (2003), pp. 595-601ArticleDownload PDFView Record in ScopusGoogle ScholarSteenholdt et al., 2014C. Steenholdt, K. Bendtzen, J. Brynskov, O.O. Thomsen, M.A. AinsworthClinical implications of measuring drug and anti-drug antibodies by different assays when optimizing infliximab treatment failure in Crohn's disease: Post hoc analysis of a randomized controlled trialThe American Journal of Gastroenterology, 109 (2014), pp. 1055-1064CrossRefView Record in ScopusGoogle ScholarStephanowitz et al., 2012H. Stephanowitz, S. Lange, D. Lang, C. Freund, E. KrauseImproved two-dimensional reversed phase-reversed phase LC-MS/MS approach for identification of peptide-protein interactionsJournal of Proteome Research, 11 (2012), pp. 1175-1183CrossRefView Record in ScopusGoogle ScholarSun et al., 2014L. Sun, A.S. Hebert, X. Yan, Y. Zhao, M.S. Westphall, M.J. Rush, ..., N.J. DovichiOver 10,000 peptide identifications from the HeLa proteome by using single-shot capillary zone electrophoresis combined with tandem mass spectrometryAngewandte Chemie (International Ed. in English), 53 (2014), pp. 13931-13933CrossRefView Record in ScopusGoogle ScholarSwaney et al., 2010D.L. Swaney, C.D. Wenger, J.J. CoonValue of using multiple proteases for large-scale mass spectrometry-based proteomicsJournal of Proteome Research, 9 (2010), pp. 1323-1329CrossRefView Record in ScopusGoogle ScholarTabernero et al., 2017J. Tabernero, A. Ohtsu, K. Muro, E. Van Cutsem, S.C. Oh, G. Bodoky, ..., C.S. FuchsExposure-response analyses of Ramucirumab from two randomized, phase III trials of second-line treatment for advanced gastric or gastroesophageal junction cancerMolecular Cancer Therapeutics, 16 (2017), pp. 2215-2222View Record in ScopusGoogle ScholarTargan et al., 1997S.R. Targan, S.B. Hanauer, S.J. van Deventer, L. Mayer, D.H. Present, T. Braakman, ..., P.J. RutgeertsA short-term study of chimeric monoclonal antibody cA2 to tumor necrosis factor alpha for Crohn's disease. Crohn's Disease cA2 Study GroupThe New England Journal of Medicine, 337 (1997), pp. 1029-1035View Record in ScopusGoogle ScholarTrenchevska et al., 2016O. Trenchevska, R.W. Nelson, D. NedelkovMass spectrometric immunoassays for discovery, screening and quantification of clinically relevant proteoformsBioanalysis, 8 (2016), pp. 1623-1633CrossRefView Record in ScopusGoogle ScholarUeda et al., 2011K. Ueda, N. Saichi, S. Takami, D. Kang, A. Toyama, Y. Daigo, ..., H. NakagawaA comprehensive peptidome profiling technology for the identification of early detection biomarkers for lung adenocarcinomaPLoS One, 6 (2011), Article e18567CrossRefGoogle ScholarUhlen et al., 2015M. Uhlen, L. Fagerberg, B.M. Hallstrom, C. Lindskog, P. Oksvold, A. Mardinoglu, ..., F. PontenProteomics. Tissue-based map of the human proteomeScience, 347 (2015), p. 1260419CrossRefGoogle ScholarUhlen et al., 2010M. Uhlen, P. Oksvold, L. Fagerberg, E. Lundberg, K. Jonasson, M. Forsberg, ..., F. PontenTowards a knowledge-based human protein atlasNature Biotechnology, 28 (2010), pp. 1248-1250CrossRefView Record in ScopusGoogle ScholarValkenborg et al., 2008D. Valkenborg, I. Jansen, T. BurzykowskiA model-based method for the prediction of the isotopic distribution of peptidesJournal of the American Society for Mass Spectrometry, 19 (2008), pp. 703-712ArticleDownload PDFCrossRefView Record in ScopusGoogle ScholarVasan, 2006R.S. VasanBiomarkers of cardiovascular disease: Molecular basis and practical considerationsCirculation, 113 (2006), pp. 2335-2362View Record in ScopusGoogle ScholarWong, 2001S.H. WongTherapeutic drug monitoring for immunosuppressantsClinica Chimica Acta, 313 (2001), pp. 241-253ArticleDownload PDFView Record in ScopusGoogle ScholarWu and Kabat, 1970T.T. Wu, E.A. KabatAn analysis of the sequences of the variable regions of Bence Jones proteins and myeloma light chains and their implications for antibody complementarityThe Journal of Experimental Medicine, 132 (1970), pp. 211-250CrossRefView Record in ScopusGoogle ScholarZeeberg et al., 2003B.R. Zeeberg, W. Feng, G. Wang, M.D. Wang, A.T. Fojo, M. Sunshine, ..., J.N. WeinsteinGoMine
- Home >
- Catalog >
- Legal >
- Warranty Deed Form >
- Special Warranty Deed >
- general warranty deed definition >
- Analysis Of Biomolecular And Cellular Systems 2011 Problem Set 1