A Complete Guide to Editing The Form 4b Factory Act
Below you can get an idea about how to edit and complete a Form 4b Factory Act hasslefree. Get started now.
- Push the“Get Form” Button below . Here you would be taken into a dashboard that enables you to carry out edits on the document.
- Choose a tool you need from the toolbar that appears in the dashboard.
- After editing, double check and press the button Download.
- Don't hesistate to contact us via [email protected] if you need further assistance.
The Most Powerful Tool to Edit and Complete The Form 4b Factory Act
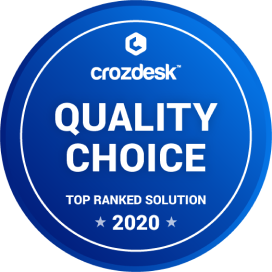
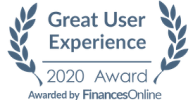
A Simple Manual to Edit Form 4b Factory Act Online
Are you seeking to edit forms online? CocoDoc has got you covered with its comprehensive PDF toolset. You can get it simply by opening any web brower. The whole process is easy and quick. Check below to find out
- go to the PDF Editor Page of CocoDoc.
- Upload a document you want to edit by clicking Choose File or simply dragging or dropping.
- Conduct the desired edits on your document with the toolbar on the top of the dashboard.
- Download the file once it is finalized .
Steps in Editing Form 4b Factory Act on Windows
It's to find a default application capable of making edits to a PDF document. Luckily CocoDoc has come to your rescue. Examine the Manual below to find out how to edit PDF on your Windows system.
- Begin by adding CocoDoc application into your PC.
- Upload your PDF in the dashboard and make edits on it with the toolbar listed above
- After double checking, download or save the document.
- There area also many other methods to edit PDF, you can check it here
A Complete Manual in Editing a Form 4b Factory Act on Mac
Thinking about how to edit PDF documents with your Mac? CocoDoc has the perfect solution for you. It enables you to edit documents in multiple ways. Get started now
- Install CocoDoc onto your Mac device or go to the CocoDoc website with a Mac browser. Select PDF file from your Mac device. You can do so by pressing the tab Choose File, or by dropping or dragging. Edit the PDF document in the new dashboard which includes a full set of PDF tools. Save the file by downloading.
A Complete Guide in Editing Form 4b Factory Act on G Suite
Intergating G Suite with PDF services is marvellous progess in technology, with the potential to simplify your PDF editing process, making it quicker and more cost-effective. Make use of CocoDoc's G Suite integration now.
Editing PDF on G Suite is as easy as it can be
- Visit Google WorkPlace Marketplace and search for CocoDoc
- install the CocoDoc add-on into your Google account. Now you are ready to edit documents.
- Select a file desired by clicking the tab Choose File and start editing.
- After making all necessary edits, download it into your device.
PDF Editor FAQ
Where does the quote "May you live in interesting times" come from?
It was first used by Sir Austen Chamberlain in 1936, and later popularized through a speech by Robert F Kennedy in 1966. The phrase “live in interesting times” dates at least to the late 19th century. The “Chinese curse” element was likely added by Sir Chamberlain as an (effective) embellishment. There is no evidence of a Chinese origin.That's the short answer, as best we know it. Longer answer (you know me…) follows. I will variously refer to it as “the quote” or simple the Curse. I will attempt to summarize the definitive history of this oddly intriguing meme.The earliest clearly identified usage of the quote is by Austen Chamberlain in a speech in March, 1936:“It is not so long ago that a member of the Diplomatic Body in London, who had spent some years of his service in China, told me that there was a Chinese curse which took the form of saying, ‘May you live in interesting times.’ There is no doubt that the curse has fallen on us.” [1]A separate source confirming that Austen Chamberlain used this quote is from Frederic R. Coudert, who also referenced 1936 use of the quote:“Some years ago, in 1936, I had to write to a very dear and honored friend of mine, who has since died, Sir Austen Chamberlain, brother of the present Prime Minister, and I concluded my letter with a rather banal remark, "that we were living in an interesting age." Evidently he read the whole letter, because by return mail he wrote to me and concluded as follows: "Many years ago, I learned from one of our diplomats in China that one of the principal Chinese curses heaped upon an enemy is, 'May you live in an interesting age.'" "Surely", he said, "no age has been more fraught with insecurity than our own present time." That was three years ago.” [2]The core part of the curse, living in interesting times, was a phrase frequently used by Austen’s father, Joseph Chamberlain, such as in this 1898 speech:I think that you will all agree that we are living in most interesting times. (Hear, hear.) I never remember myself a time in which our history was so full, in which day by day brought us new objects of interest, and, let me say also, new objects for anxiety. (Hear, hear.) [3]Austen Chamberlain was British Foreign Secretary in 1924 to 1929, thus 10 years prior to the above letter exchange. Thus it is possible that the “curse” has a genuinely Chinese origin, but only very vague comparables have been identified, and no direct evidence has been found. Given the vagaries of human memory, and our equally human tendency to embellish, it could just as easily simply be an invention of Chamberlain’s, and he is lending credence to it with reference to a nebulous (“many years ago … I learned”) origin in exotic China, something that he can specifically can lend credence to from his diplomatic career. Bear in mind that in 1936, he was aged 73, and this was inked in a personal letter.Sir Austen ChamberlainIf indeed coined by him, and at this time, it was not in a neutral setting - it is distinctly political and polemic in nature, and was being used by Austen Chamberlain in the context of arguing for a rapid rearmament of Britain in the face of the threat of Nazi Germany.Barring evidence to the contrary, then, I would posit that Sir Austen Chamberlain invented the “curse” in 1936 or shortly prior to that, in the course of his political speechmaking and letter writing.[[A few curious asides (skip this paragraph unless you enjoy segues): Austen Chamberlain was Joseph Chamberlain’s first-born son from his first marriage (his mother died in childbirth). Neville Chamberlain, who was to become the fateful prime minister, was his second son (the first child of his second marriage). Austen received the Nobel Peace Prize in 1926 for his work in reconciling France and Germany after the first world war. It was for being part originator of the Locarno Act and thus “for work aimed at ensuring peace between the arch-rivals Germany and France”. The Nazi rise to power in the 1932 elections (and Hitler becoming Chancellor in January of the next year) let to Germany beginning a (partially covert) effort to re-arm, which prompted both Austen and his half-brother Neville to advocate for rearming. Austen died just weeks before Neville became prime minister, in 1937. Neville is unjustly treated in the popular mind by the focus on his “peace in our time” quote - the reality is that he pushed for modernization of the British industry and accelerated re-armament, as much as the public politic could accept before 1940. His policies of “Rationalisation” as Chancellor of the Exchequer in 1931–1937 helped modernize British factories, a key factor in winning the fabled Battle of Britain in 1940 - contrary to the popular rendition of the aerial battle, Britain won largely because they could build airplanes faster than the Germans could (*).]]So that would seem to settle (in my mind at least) the “creation” question of the quote. Related is: how did it come to spread? Here we have to get a little speculative. At the time, from 1936 onwards, the quote appears in print several times in the late 1930s, early 1940s, but not in any manner that would seem to cause it to spread very far. Quote Investigator meticulously documents the known cases [4]. Presumably, somebody picked up the proverbial baton.One “thread” of usage continued through the political sciences, as well as in France, with the Curse continuing to occasionally show up in political speeches or writings, in the 1940s and 1950s [4B]. But these were obscure. The candidate for having the largest impact is Eric Frank Russell [5].Mr Russell was born in 1905 in Berkshire, England, and his father was an instructor at the Royal Military College. He became a fan of science fiction in 1934, his first short story was in 1937 and his first novella in 1939, after which he served in the war.Thus in 1936, Mr Russell was in his late 20s, and a budding science fiction writer. With his father an instructor at RMC, and him of a literary bent, it would seem likely that he would come across the Curse, either from reading it, or from his father having read it, or indeed either of them hearing it from Austen Chamberlain directly.Our evidence for this is that the Curse disappears from print after World War 2. Understandably - things got perhaps a little too interesting. It next appears in a science fiction story by a certain Duncan H. Munro:"For centuries the Chinese used an ancient curse: 'May you live in interesting times!' It isn't a curse any more. It's a blessing. We're scientific and civilized. We've got so many rights and liberties and freedoms that one can yearn for chains for the sheer pleasure of busting them and shaking them off. Reckon life would be more livable if there were any chains left to bust." [6]This was published in 1950. “Duncan H. Munro” is a pseudonym for Eric Frank Russell. It is the earliest use of the Curse in fiction, and it’s the earliest use that one would expect to have a broader readership as well.At this point, the Curse enters the Science Fiction vernacular. The magazine in question, Astounding Science Fiction, had its heyday in the period 1939–1960. The year in question (1950) was particularly strong - each issue had 4–6 stories, and other writers that year included Isaac Asimov (including parts that would become The Foundation series), A.E. van Vogt, L. Ron Hubbard, Poul Anderson, and L. Sprague de Camp. Eric Russell is the first to use the Curse, but it gets picked up by none other than Arthur C. Clarke, who is likely to have read anything published in that magazine in 1950 (he himself published several short stories in it, the last of which in September 1949, “Hide and Seek”).“[It is] never easy to live in an age of transition - indeed, of revolution. As the old Chinese curse has it: “May you live in interesting times,” and the twentieth century is probably the most “interesting” period mankind has ever known.” [7]Mr Clarke was an outsize thinker about space and the future in 1965. President John F. Kennedy had announced the goal of going to the moon in 1961. Stanley Kubrick had met with Clarke in 1964 to discuss collaborating on a film, loosely based on Clarke’s short story The Sentinel from 1948. The novel they wrote together based on the short story was “2001”, with both the movie and the book coming out in 1968. The story and the movie, of course, circles around a discovery of the moon.The significance will shortly be clear. Because the seminal moment that made the Curse a wide-spread wisdom was the use by Robert F. Kennedy in 1966:For the fortunate among us, the fourth danger is comfort, the temptation to follow the easy and familiar paths of personal ambition and financial success so grandly spread before those who have the privilege of education. But that is not the road history has marked out for us. There is a Chinese curse which says "May he live in interesting times." Like it or not we live in interesting times. They are times of danger and uncertainty; but they are also more open to the creative energy of men than any other time in history. And everyone here will ultimately be judged-will ultimately judge himself-on the effort he has contributed to building a new world society and the extent to which his ideals and goals have shaped that effort. [8]Now, there are two ways the quote could have made it here. First one is most obvious - Robert Kennedy gave the speech in 1966, and Arthur C. Clarke had published “Voices from the Sky” in 1965, everybody was talking about the Apollo Moon program, and Clarke was one of the most influential public thinkers about the significance of the Space Race.Another possibility is that it was contributed by his speech writers. The primary authors of Kennedy's speech are believed to have been Richard Goodwin and Adam Walinsky. Walinsky would have been age 13 when the original Eric Russell story was published. Goodwin is several years older, he graduated from Harvard in 1952. It would seem quite possible that young Adam leveraged some youthful light reading. Or perhaps it was both - an adult Mr Walinsky may well have read Clarke’s 1965 book, now aged 29, and thus for the second time come across the Curse in his reading life. As one of the greatest speech writers of his generation, presumably Walinsky is a prolific reader with excellent memory [9].Finally: this particular 1966 speech by Mr Kennedy was not just any speech. Later known as the “Ripple of Hope” speech, it was a highly influential visit, evoking the struggle for racial equality in both the United States and South Africa, and is viewed by historians as the greatest speech of his career [10]. President Obama visited again in 2013, remind the world of the RFKs contributions.Senator Robert F. Kennedy meeting with anti-apartheid activist Chief LuthuliSo there you have it - from Chamberlain to Kennedy. Perhaps I am being too fanciful and romantic, but that’s the long version I chose to believe in: invented by Austen Chamberlain to argue for arming against Nazi Germany, ascribed a mythic origin in the Orient, and eventually used by Robert F. Kennedy to argue for the fall of Apartheid. Given the towering intellects of RFK and his speech writers, I wouldn’t be entirely surprised if it was very deliberate - that one of them knew full well that the phrase had been used by Austen. But it could also be one of History's many delicious ironies.(*) Seque within the segue: The conventional wisdom is that Britain won because when their pilots were shot down, they could hop into a new airplane, whereas the Germans could not. But this argument presupposes that there’s an actual airplane to jump into, and that was the real bottleneck - industrial production. The British at the time emphasized individual pilot heroism, which was obviously substantial, but that was not a material advantage over the Germans. In analysis of production numbers after WW2, we now know that the British were capable of building airplanes faster than they were losing them, and the Germans could not, something the Germans presumably figured out and left them no choice but to disengage. Also, one can’t help but wonder if Churchill, who was a politically astute opponent of Neville Chamberlain, was only too happy to downplay the significance of Britain’s post-depression economic policies, an argument that couldn’t be defended by Neville since by then he had passed away. I include both the segue and the double-segue because, to me at least, it’s significant that the curse was invented in this specific historical context. And I suspect that this was not lost Eric Russell.[1] 1936 March 21, The Yorkshire Post, Lesson of the Crisis: Sir A. Chamberlain’s Review of Events, Quote Page 11, Column 7, Leeds, West Yorkshire, England. (British Newspaper Archive). This citation was discovered by Bonnie Taylor-Blake, contributing to http://QuoteInvestigator.com[2] 1939 May, Proceedings of the Academy of Political Science, Volume XVIII, The Preservation of Democracy - America’s Preparedness, edited by John A Krout, page 269.[3] 1898 January 21, The Western Daily Press, Mr. Chamberlain at Liverpool: A Series of Speeches, Patriotism Still a Live Force, Page 8, Column 3, Bristol, England. (British Newspaper Archive)[4] 2015 December, from the web: May You Live In Interesting Times.[4B] Documented by the aforementioned contributors to Quote Investigator, as well as Fred R. Shapiro.[5] From the web: Sir Austen Chamberlain - Facts.[5] Research results pinpointing roots in Joseph and Austen Chamberlain were done by contributors to Quote Investigator and Taylor-Blake as noted above. Research resulting in Russell and attendant Kennedy speechwriter connection was done by yours truly in 2002 (not published).[6] 1950 April. “U-Turn” by Duncan H. Munro, published in Astounding Science Fiction, page 137.[7] 1965. “Space and the Spirit of Man” appeared in “Voices from the Sky” published in 1965 by Harper & Row, New York), Published by St. Martin’s Press, New York.[8] 1966, June. Robert F. Kennedy, University of Cape Town, South Africa, N.U.S.A.S. "Day of Affirmation" Speech June 6th, 1966[9] I have tried to reach out to Mr Walinsky to see if he can recall these connections, but unfortunately I have not heard back from him. He is still professionally active, so, if anybody reading this knows him, give him a poke.[10] See for example “RFK in the Land of Apartheid: a Ripple of Hope”, on PBS (from the web RFK in the Land of Apartheid | PBS Programs | PBS) as well as the film’s website at: A Ripple of Hope
Is capitalism more 'natural' to human behavior than socialism and communism?
Short answer: No. Capitalism is not natural to human beings. In fact, it is antithetical to human beings and this is why there are so many mental disorders and anxiety and depression in modern society.A bit longer answer: Human beings have evolved as hunter gatherers, living in small groups, sharing, working together, and not competing against each other. Selfishness was punished by the group and selected against in natural selection, as those ostracized would be less likely to reproduce. The capitalist system embodies characteristics which are not natural to human nature, thus leading to mental and emotional problems in individuals. Further, the capitalist system requires selfishness and competition for basic survival. Individual morality is challenged by basic survival considerations, and the desire to survive is stronger in most humans. Psychopaths and narcissists tend to become the leaders of a psychopathic system, owned by psychopathic systems—corporations.Detailed answer:No. I am always surprised when I hear this. Some say that humans are naturally selfish, greedy, looking to gain advantage over others, and that capitalism embodies these realities. They believe that if everyone acts in their own best economic interest then they will form businesses to make money, which will benefit society. They believe that people are naturally lazy, so if they are given “free stuff” they won’t work. People need the threat of homelessness or starvation to be motivated.This view of human nature is common among the political right. They are wrong. Human beings have lived as hunter gatherers for hundreds of thousands of years until 12,000 years ago. During this period people began to live in village and developed agriculture. Specialization in occupations developed, as did the use of money. Social classes came into being.The hunter gatherers lived under primitive communism. They lived in societies which were moneyless, stateless, and classless. They managed themselves by the men hunting in groups and the women foraged for berries and other things while watching the children. If one person didn’t catch something, someone who did shared with him. And vice versa. Nothing could be more communist than this. The human brain evolved for this lifestyle. In fact cooperation is an essential aspect of human psychology. Punishing those who are selfish and don’t return favors is built into human psychology. Cooperation is the norm. Without it people in difficult climate conditions would have died out. Selfish people would be more likely to be ostracized, which could mean death. Natural selection worked against selfishness. But this doesn’t mean that humans are creatures ruled by pure altruism. Rather, humans are adaptable. In prosocial societies with cooperation they will cooperate. In selfish societies they will be more cautious and less generous. The human brain is incredibly adaptable.The problem with capitalism is that selfishness is an inherent part of the system. Altruism will leave you at a disadvantage against those who embody selfishness. Competition also increases these demands. For example, if a CEO decides to be really nice and not move the auto factory away from Detroit corporate profits will decline as American workers make more money than Mexican workers. Shareholders are sometimes large retirement funds. The managers of funds are expected to select stocks which are performing well. This is because those in retirement rely upon the income for survival. So the board of directors starts to get pressure from below. They put pressure on the CEO. The reality becomes that if he refuses to move the factory he will be fired and the next CEO will move it. So no amount of individual goodness is going to change the system.Not surprisingly there are many narcissistic and psychopathic individuals that run large corporations and are political leaders. Corporations are psychopaths. They are amoral, are dedicated to one single goal—getting money. Everything else is secondary. Of course psychopaths do well in this atmosphere, because they are willing to do whatever is needed to succeed. Your average person wouldn’t be willing to become CEO and lay people off before Christmas, cut retirement benefits, or any number of things required of CEO’s. But this does not mean that human nature is this way.It is short sighted to assume that the values of the economic system in which you live are “human nature.” During feudalism they believed that was human nature. They even took it further and claimed that monarchs had a divine right to rule, and that was God’s intention. Was that human nature? No.Human nature is the production of evolution in the context of human beings living as hunter-gatherers. And this meant cooperation.Interestingly, cooperation is also present in non human lifeforms, and evolution has designed strategies to punish non cooperators to ensure the survival of the group.This paper details the mechanisms behind cooperation and human behavior, as well as examples of other species.The Problem of CooperationDefinitionsActor: the focal individual performing a behaviour.Altruism: a behaviour that is costly to the actor and beneficial to the recipient. Cost and benefit are defined on the basis of the lifetime direct fitness consequences of a behaviour.Cheaters: individuals who do not cooperate or who cooperate less than their fair share, but are potentially able to gain the benefit of others cooperating.Cooperation: a behaviour that provides a benefit to another individual (recipient), and the evolution of which has been dependent on its beneficial effect for the recipient.Direct fitness: the component of fitness gained from producing offspring; the component of personal fitness due to one's own behaviour.Greenbeard: a hypothetical gene that causes in carriers both a phenotype that can be recognised by conspecifics (a ‘green beard’) and a cooperative behaviour towards conspecifics who show a green beard.Inclusive fitness: “the effect of one individual's actions on everybody's numbers of offspring […] weighted by the relatedness [10]; the sum of direct and indirect fitness; the quantity maximised by Darwinian individuals.Indirect fitness: the component of fitness gained from aiding related individuals.Kin selection: process by which traits are favoured because of their beneficial effects on the fitness of relatives.Mutual benefit: a benefit to both the actor and the recipient.Mutualism: two-way cooperation between species.Recipient: an individual who is affected by the behaviour of the focal individual.Relatedness: a measure of the genetic similarity of two individuals, relative to the average; the statistical (least-squares) regression of the recipient's breeding value for a trait on the breeding value of the actor.A behaviour is cooperative if it provides a benefit to another individual and if it has evolved at least partially because of this benefit [1]. Such behaviours pose a problem to evolutionary theory because — all else being equal — they would reduce the relative fitness of the performer of that behaviour and hence be selected against [2](Figure 1). To give a specific example, consider the star of many a nature documentary, the meerkat. Meerkats generally live in groups of up to 30 adults with their young. The adults of a group can be divided into the dominant male and female, who do most of the breeding, and the subordinates, who help the dominants raise their offspring [3]. When one of these subordinates has found a tasty scorpion, why should it hand it over to one of the offspring produced by the dominant pair? How can we reconcile this behaviour with selfish interests, even at the level of the gene, and the Darwinian struggle for survival and reproduction in the natural world?Download high-res image (86KB)Download full-size imageFigure 1. The problem of Under Construction the absence of one of the mechanisms discussed in this review, natural selection favours selfish individuals who do not cooperate. Consider a population of cooperators (‘C’) in which an uncooperative, selfish cheater (‘S’) arises through mutation or migration. In a mixed population, the selfish cheater benefits from the cooperative behaviour of the cooperators, without paying the cost. Consequently, the selfish cheater has a higher fitness than the cooperators and spreads through the population, despite the fact that this leads to a decline in mean fitness. (Redrawn after [104].)This problem also applies to economics and human morality, with a famous example being the ‘tragedy of the commons’ [4]: imagine a number of shepherds, each deciding how many sheep to keep on a shared pasture. The addition of extra sheep will have both a benefit and a cost. The benefit is that the shepherd will gain from extra sheep. The cost is potential overgrazing, which can damage the pasture. However, whilst our focal shepherd gains all of the benefit, he pays only a fraction of the cost, which is shared between all of the shepherds. Consequently, the individual shepherd has more to gain than to lose from adding extra sheep. The tragedy is that — as a group — all the shepherds would benefit from grazing less sheep. Such cooperation, however, is not stable, because each individual can gain by selfishly pursuing their own interests.Most attention on the problem of cooperation (see Box 1 for glossary) has been focused on interactions between animals. However, the same problem occurs at all levels of biological organisation 2, 5, 6, 7. The very existence of multicellular organisms relies upon cooperation between the eukaryotic cells that make them up. The mitochondria upon which these eukaryotic cells rely were once free-living prokaryotic cells but now live cooperative lives. Separate genes, which make up the genome, cooperate in what has been termed the ‘parliament of the genes’ [8]. The tree of life is dominated by single-celled microorganisms that appear to perform a huge range of cooperative behaviours [9]. For example, the growth and survival of bacteria depend upon excreted products that perform a variety of functions, such as scavenging nutrients, communication, defence and movement. The benefits of such extracellular products can be shared by neighbouring cells and hence they represent a ‘public good’ that is open to the problem of cooperation [9]. Almost all of the major evolutionary transitions from replicating molecules to complex animal societies have relied upon solving the problem of cooperation being solved [7].The SolutionsAs cooperation is in evidence throughout the natural world, there must be a solution to the problem. Theoretical explanations for the evolution of cooperation (or any behaviour) are broadly classified into two categories: direct fitness benefits or indirect fitness benefits 2, 10, 11, 12 (Figure 2). This follows from Hamilton's [2]insight that individuals gain inclusive fitness through their impact on the reproduction of related individuals (indirect fitness effects) as well as through their impact on their own reproduction (direct fitness effects) (Figure 3). The importance of Hamilton's work cannot be overstated — it is one of the few truly fundamental advances since Darwin in our understanding of natural selection.Download high-res image (197KB)Download full-size imageFigure 2. A classification of the explanations for cooperation.Direct benefits explain mutually beneficial cooperation, whereas indirect benefits explain altruistic cooperation. Within these two fundamental categories, the different mechanisms can be classified in various ways 1, 12, 55, 72. These possibilities are not mutually exclusive; for example, a single act of cooperation could have both direct and indirect fitness benefits or interactions with relatives could be maintained by both limited dispersal and kin discrimination. Our dividing up of conditional enforcement strategies is for illustration only, a detailed discussion is found elsewhere [72].Download high-res image (171KB)Download full-size imageFigure 3. Inclusive fitness and cooperation.Inclusive fitness is the sum of direct and indirect fitness [2]. Social behaviours affect the reproductive success of self and others. The impact of the actor's behaviour (yellow hands) on its reproductive success (yellow offspring) is the direct fitness effect. The impact of the actor's behaviour (yellow hands) on the reproductive success of social partners (blue offspring), weighted by the relatedness (r) of the actor to the recipient, is the indirect fitness effect. Inclusive fitness does not include all of the reproductive success of relatives (blue offspring), only that which is due to the behaviour of the actor (yellow hands). Also, inclusive fitness does not include all of the reproductive success of the actor (yellow offspring), only that which is due to its own behaviour (yellow hands).A cooperative behaviour yields direct fitness benefits when the reproductive success of the actor, who performs the cooperative behaviour, is increased. Cooperative behaviours that benefit both the actor and the recipient(s) of the behaviour are termed ‘mutually beneficial’ [1]. A cooperative behaviour can be explained by indirect fitness benefits if it is directed towards other individuals who carry the gene for cooperation [2]. This is usually termed ‘kin selection’ [13], because the simplest and most common way this could occur is if cooperation is directed at relatives, who share genes from a common ancestor [11]. By helping a close relative reproduce, an individual is still passing copies of its genes on to the next generation, albeit indirectly. Cooperative behaviours that are costly to the actor and beneficial to the recipient are termed ‘altruistic’ [2] and can only be explained by indirect fitness benefits. A key point here is that we are considering the average consequences of a behaviour and not the consequences of every single instance.Kin Selection and Indirect Fitness BenefitsHamilton's inclusive fitness (kin selection) theory shows how altruistic cooperation can be favoured between relatives. This is encapsulated in a pleasingly simple form by Hamilton's rule [2], which states that a behaviour or trait will be favoured by selection, when rb−c>0, where c is the fitness cost to the actor, b is the fitness benefit to the recipient, and r is their genetic relatedness. Putting this inequality into words, altruistic cooperation can therefore be favoured if the benefits to the recipient (b), weighted by the genetic relatedness of the recipient to the actor (r), outweigh the costs to the actor (c). This follows from inclusive fitness theory because −c represents the direct fitness consequences of a social behaviour and rb the indirect fitness consequences. Hamilton's rule predicts greater levels of cooperation when r or b are higher and c is lower.Explanations for cooperation based on indirect fitness benefits, i.e. kin selection, require a sufficiently high genetic relatedness (r) between interacting individuals. The coefficient of relatedness (r) is a statistical concept, describing the genetic similarity between two individuals, relative to the average similarity of all individuals in the population [11]. Hamilton [2] suggested two possible mechanisms through which a high relatedness could arise between social partners: kin discrimination and limited dispersal.Kin Selection and Kin DiscriminationThe first mechanism for generating sufficiently high relatedness to make kin selection viable is kin discrimination, when an individual can distinguish relatives from non-relatives and preferentially direct aid towards them (nepotism) [2]. This has been demonstrated in several cooperatively breeding vertebrates, such as long-tailed tits [14], where individuals that fail to breed independently help at the nest of closer relatives [14] (Figure 4A). In this species, individuals distinguish between relatives and non-relatives on the basis of vocal contact cues, which are learned from adults during the nesting period (associative learning) [15]. This leads to a situation in which individuals tend to help relatives whom they have been associated with during the nestling phase.Download high-res image (168KB)Download full-size imageFigure 4. Kin discrimination.(A) Kin discrimination in long-tailed tits. 96% of helpers prefer to help at nests containing related chicks when they have the choice of where to invest their efforts. With permission from [14]. (B) Kin discrimination in the unicellular slime mold Discoideum purpureum (social amobae) [18]. A scatter plot shows the proportion of fluorescently labelled spores in fruiting bodies when two isolates are placed together at equal proportions and one is fluorescently labelled (bold). There is a greater variance in the experimental treatment where the two isolates are different lineages (X and Y), than in the control treatment where the isolates are the same lineage. This shows that individuals preferentially form fruiting bodies with members of their own lineage. With permission from [18].Kin selection theory also explains the variation in the level of kin discrimination across species [16]. In contrast to the long-tailed tits, other cooperatively breeding vertebrates, such as meerkats [17], do not show kin discrimination when helping. The advantage of kin discrimination will be positively correlated with the extent of the benefit (b) provided by helping. In the extreme, if a supposedly ‘helping’ behaviour provides little or no benefit to its recipients, then there is little or no advantage in directing it towards closer relatives. This leads to the prediction that the extent of kin discrimination should be greater in species where a greater fitness benefit is derived from receiving help — a pattern indeed observed across cooperatively breeding vertebrate species [16] (Figure 5). Overall, the benefit that helping brings to the recipient can explain 42% of the variation in the extent of kin discrimination across species.Download high-res image (113KB)Download full-size imageFigure 5. Kin discrimination and the benefit of helping.Across cooperatively breeding bird and mammal species, helpers are more likely to discriminate in favour of relatives when the amount of help they provide increases the survival of offspring to the following year. The extent to which individuals preferentially help closer relatives (kin discrimination) is plotted against the benefit of helping. The significant positive relationship between these two variables is predicted by kin selection theory. The illustration shows the laughing kookaburra, a species which does not show kin discrimination in its helping behaviour. Modified with additional data points from [16].Kin discrimination has also been found in species that are not usually thought of from a social perspective. Dictyostelium purpureum is a unicellular slime mould found in forest soils [18]. When starved of its bacterial food source, the cells of this species aggregate in thousands to form a multicellular, motile ‘slug’. Slugs migrate to the soil surface, where they transform into a fruiting body composed of a stalk structure holding aloft a ball of spores. The non-viable stalk cells are sacrificed to aid the dispersal of the spores. This requires explanation because cooperative cells that form stalk cells could be exploited by cheaters who avoid the stalk and instead migrate to form spores in the fruiting body. Kin selection offers a potential solution to this problem, because stalk cells could gain an indirect fitness benefit from helping relatives disperse. This suggests that it would be advantageous for the individual amoebae to preferentially form a slug with relatives. Indeed, kin discrimination during slug formation has recently been observed in D. purpureum[18]. Specifically, when two lineages are mixed and allowed to form slugs on agar plates, they discriminate to the extent that the average relatedness in fruiting bodies increases to a value of 0.8, as opposed to the expected value of 0.5 (Figure 4B).Kin Discrimination CuesKin discrimination can occur through the use of environmental or genetic cues [19]. The most common mechanism appears to involve environmental cues, such as prior association or shared environment, as in long-tailed tits and a range of other animals from humans to ants 20, 21. In contrast, in the case of the slime mould some genetic cue of relatedness is likely to be involved — also termed ‘kin recognition’, ‘genetic similarity detection’, ‘matching’ or ‘tags’. In order to detect genetic similarity, an individual must have some cue that is genetically determined — such as the cuticular hydrocarbon profile of an insect [22], or the odour produced by scent glands in a mammal [23] — and a ‘kin template’ for comparison [19]. This kin template could be determined by the individual's own genotype or cues (‘self-matching’) and/or through learning the cues of its rearing associates [23].Kin discrimination based on genetic cues is often unlikely to be evolutionarily stable. The reason is that recognition mechanisms require genetic variability (polymorphism) in order to provide a cue. However, individuals with common genetic variants would be more likely to be helped, and thus more common genes would be driven to fixation [24]. Consequently, kin discrimination is, as it were, its own worst enemy, eliminating the genetic variability that it requires to work. Thus, kin discrimination based on genetic cues is often not found where it might be expected 25, 26, 27, 28. In cases where kin discrimination based upon genetic cues has been observed, it can usually be argued that there is some other selective force maintaining variability at the recognition loci, such as host-parasite co-evolution in the major histocompatibility complex (MHC) of vertebrates [24]. Cue diversity may also be maintained if there is limited dispersal, such that interactants tend to be relatives anyway 24, 29, as is likely to be the case with the slime mould discussed above.Green BeardsIndirect fitness benefits will also be obtained if cooperation is directed towards non-relatives who share the same cooperative gene 2, 30. Dawkins [31] illustrated this with a hypothetical example of a gene that gave rise to green beards, while simultaneously prompting individuals with green beards to preferentially direct cooperation towards other green-bearded individuals. This mechanism can also occur without a visible tag — for example, if the cooperative gene also caused some effect on habitat preference that led to individuals who carried that gene settling together [30]. Consequently, although this mechanism is usually termed a ‘greenbeard’, it more generally represents an assortment mechanism, requiring a single gene — or a number of tightly linked genes — that encodes both the cooperative behaviour and causes cooperators to associate [12].Greenbeards are likely to be rare, because cheaters that display the green beard, or assorting behaviour, without also performing the cooperative behaviour, could invade and overrun the population. Furthermore, in the absence of relatedness over the whole genome, the altruistic greenbeard would be in conflict with genes elsewhere in the genome, where there would be strong selection for suppression of the greenbeard 32, 33. One of the few cases where a cooperative greenbeard occurs is in the slime mould Dictyostelium discoideum, which forms fruiting bodies in a very similar way to D. purpureum. In. D. discoideum, individual amoebae with the csa cell-adhesion gene adhere to each other in aggregation streams and cooperatively form fruiting bodies at the exclusion of csa mutants [34]. It is perhaps not surprising that greenbeards should be rare, given that the idea was not developed as a theory to explain altruism, but as a thought experiment to show that genetic relatedness — rather than genealogical relationship per se — is the key to kin selection.Kin Selection and Limited DispersalLimited dispersal (population viscosity or structure) can generate high degrees of relatedness between interacting individuals because it will tend to keep relatives together 2, 5. In this case, altruism directed indiscriminately at neighbours could be favoured, because those neighbours are more likely to be relatives. This mechanism has the potential to be important in a wide range of organisms because it does not require any mechanism of kin discrimination. Instead, all that is required is that the level of cooperation evolves in response to the mean relatedness between individuals who tend to interact by chance.The predicted role of limited dispersal has been supported by an experimental evolution study on cooperation in bacteria [35]. Many bacteria release siderophore molecules to scavenge for iron. These represent a cooperative public good: they are costly to the individual to produce, but iron bound to siderophores can be taken up by any cell, providing a benefit to other individuals in the locality. When populations of the bacterium Pseudomonas aeruginosa containing a mixture of a wild-type strain that produces siderophores and a cheater mutant that does not were maintained in conditions that led to relatively high or low relatedness [35], the cooperative wild-type strain out-competed the cheater mutant strain only under conditions of relatively high relatedness (Figure 6). More generally, limited dispersal is likely to be important for maintaining a range of public goods produced by bacteria to help with the gathering of resources, growth and reproduction [9].Download high-res image (132KB)Download full-size imageFigure 6. Relatedness, competition and http://cooperation.An experimental study on cooperative siderophore production in the bacteria Pseudomonas aeruginosa has shown how selection for cooperation is influenced by relatedness and the extent of competition between relatives [35]. The proportion of cooperative individuals who produce siderophores is plotted against time. The different lines represent relatively high (solid lines) and low (dashed lines) relatedness. The different symbols represent relatively low (circle) and high (triangle) amounts of competition between relatives. Cooperation is favoured by higher relatedness and lower competition between relatives. With permission from [35].Limited Dispersal and Kin CompetitionAlthough limited dispersal can favour cooperation, it will not necessarily do so [36]. The problem is that, although limited dispersal can bring relatives together to cooperate, it can also keep them together to compete 30, 37. This competition between relatives can reduce or even completely remove selection for cooperation between relatives. One way of thinking about this is that the concomitant competition reduces the benefit (b) of helping relatives [11]. In the extreme case, there is no point helping a brother, if their increase in fitness comes at the cost of another brother's reproductive success [38]. In the simplest possible scenario, the effects of increased relatedness and increased competition exactly cancel out, such that limited dispersal has no influence on the evolution of cooperation [39]. However, a number of factors, which are likely to be biologically important, can reduce the competition between relatives and hence allow limited dispersal to favour cooperation [36]. For example, when cooperation allows population expansion (as with bacterial public goods), when relatives tend to disperse together (‘budding viscosity’) or when the lifecycle involves a period of interaction with close relatives followed by a period of dispersal before competition (‘alternating viscosity’) 39, 40, 41.Although it has received little empirical attention [38], some experimental support for how competition between relatives can select against cooperation between relatives comes from the experiment with P. aeruginosa on siderophore production described above [35]. Relatively low competition between relatives was induced by allowing more productive groups to make a greater contribution to the next generation, thereby minimising local competition within groups. Relatively high competition between relatives was obtained by making all groups contribute equally to the next generation and hence making local competition between groups more important. As predicted by theory, the cheater mutant strain was able to spread to a higher proportion of the population when there was greater competition between relatives (Figure 6). More recently, it has also been realised that local competition for resources not only selects against cooperation, but it can select for individuals to spitefully harm less related individuals [42], such as when the soldier larvae of polyembryonic wasps attack and kill larvae to which they are less related 43, 44.Don't Forget b and cThere is often an overemphasis — both conceptually and empirically — on the importance of the relatedness term (r) in Hamilton's rule and a corresponding neglect of the benefit (b) and cost (c) terms 45, 46. To some extent, this is the case because genetic similarity can be measured more easily than components of fitness. However, focusing too strongly on r can lead to misunderstanding and confusion, because variation in b and c can be equally important. One of the clearest cases of such confusion arises from a consideration of why eusociality has evolved so many times in the haplodiploid Hymenoptera — the ants, bees and wasps. Hamilton 2, 5originally suggested that this was the case because their haplodiploid genetics leads to female workers being more related to their sisters (r = 0.75) than to their offspring (r = 0.5) and hence provided a relatedness benefit that predisposed them to the evolution of cooperation. However, it was later shown that this higher relatedness to sisters was exactly cancelled by a lower relatedness to brothers (r = 0.25) relative to sons (r = 0.5) [47]. Consequently, it has long been assumed that the high frequency of eusociality in the Hymenoptera is due to factors that provide a high benefit-to-cost-ratio (b/c), such as nest building, stinging and common parental care 48, 49. However, the misconception that kin selection only predicts that r is important still arises [50], whereas in reality, it predicts that r, b and c are important [51].The importance of b has been discussed above with respect to selection for kin discrimination and the benefit of helping [16] (Figure 5), and how selection for cooperative siderophore production is reduced by local competition between relatives [35] (Figure 6). An elegant example of the importance of the cost of helping (c) is provided by field experiments on the hairy-faced hover wasp [52]. In nests of this species, one to ten related females form an orderly queue to reproduce. The dominant female lays the eggs and the subordinate females forage and tend the young. High-ranked subordinates were experimentally removed from the nest, hence moving the remaining subordinates to a higher position in the queue. This increases the relative cost of helping, because it is more likely that the subordinate will succeed to the breeding position and so energy expended on foraging could reduce resources available for breeding, as well as decreasing the likelihood of surviving to gain the breeding position. As predicted by kin selection theory [53], when individuals were moved up the queue, they reduced the number of foraging trips [52]. This illustrates that, as the cost of helping increased, individuals cooperated less.Direct Fitness BenefitsThe evolution of cooperation does not only depend upon kin selection and indirect fitness benefits — cooperation can also provide a direct fitness benefit to the cooperating individual [54]. In this case, cooperation is mutually beneficial and not altruistic [1]. We divide the direct fitness explanations for cooperation into two categories (Figure 2). First, cooperation may provide a benefit, as a by-product, or automatic consequence, of an otherwise ‘self-interested’ act 45, 55. For example, cooperation could lead to an increase in group size, which increases the survival of everyone — including the individual who performs the cooperative behaviour — due to larger groups being better at avoiding predators or competing with other groups [56]. Second, there may be some mechanism for enforcing cooperation by rewarding cooperators or punishing cheaters [57]. It can also be useful to distinguish enforcement mechanisms that are behaviourally inflexible from those that are adjusted conditionally in response to the level of cooperation. In the latter case, the benefit to the actor depends upon the recipient adjusting their behaviour towards the actor in response to the actor's behaviour.By-Product BenefitsCooperation may provide some automatic benefit without enforcement. One way this could occur is if members of a group have some shared interest in cooperation. In many cooperatively breeding vertebrates — such as meerkats — a larger group size can provide a benefit to all the members of the group through an increase in survival, foraging success or the likelihood of winning conflicts with other groups [58]. In such a case, subordinate individuals can be selected to help rear offspring that are not their own, in order to increase group size — a process termed ‘group augmentation’ [56]. Selection for such helping is further increased if there is a chance that the subordinate will obtain dominance in the group at some later point, because they would then have a larger number of helpers themselves. The advantages of group augmentation would be greatest for the sex that is most likely to remain and breed in the natal group, which provides an explanation for why the level of helping is greatest in that particular sex for birds and mammals [59]. Similar benefits of increased individual success in larger groups have been suggested to be important in other cooperative organisms, such as ants and social spiders 60, 61. Things can get more complicated if the individuals in the group are related, because then by-product benefits can at the same time provide indirect fitness benefits, either because the actor helps a relative [56] or because the by-product benefits are shared with relatives [62].Direct fitness benefits also play a role in cooperative breeding in the wasp Polistes dominulus[63]. In this species, colonies are initiated by one or a few foundress females that form a dominance hierarchy with the dominant laying most of the eggs and the subordinates carrying out most of the more risky foraging. It was found that 35% of subordinates were unrelated to the dominant female. These subordinates gain significant direct fitness benefits from staying and helping on the nest because dominants suffer an appreciable mortality, and so there is approximately a 10% chance that a subordinate will have become the dominant in the group by the time the workers emerge [63]. It is unlikely that subordinates are merely helping non-relatives by accident, because there is sufficient between-individual variation in cuticular lipids to allow subordinates to distinguish relatives from unrelated nest-mates [64]. Thus, selection could have acted to reduce or remove cooperative behaviour when subordinates form a colony with non-relatives.Enforced CooperationCooperation can be enforced if there is a mechanism for rewarding cooperators or punishing cheaters 54, 57, 65. Trivers [54] emphasised that cooperation could be favoured in reciprocal interactions with individuals preferentially aiding those that have helped them in the past. In this case, cooperation provides a direct fitness benefit, as it is only favoured if the short term cost of being cooperative is outweighed by the long term benefit of receiving cooperation 1, 12. Trivers termed this ‘reciprocal altruism’, but because it provides a direct fitness benefit, it is mutually beneficial and not altruistic. Consequently, reciprocity [66] or reciprocal cooperation [65] are more appropriate terms [1]. Here, we use the term reciprocity to refer to cooperation preferentially directed at cooperative individuals, either directly (‘help those that help you’) or indirectly (‘help those that help others’) 54, 67, 68.Although such reciprocity has attracted a huge amount of theoretical attention, it is thought to be generally unimportant outside of humans 69, 70, 71. Our use of the term ‘reciprocity’ differentiates it from other cases of enforcement that also rely on behavioural flexibility and that have been variously termed ‘punishment’, ‘policing’, ‘sanctions’, ‘partner-switching’ and ‘partner choice’ 1, 55, 57, 72 (Figure 2). It is beyond the scope of this review to sort the relationships between these different mechanisms [72]. In some cases, the term ‘reciprocity’ is used more generally to cover all these instances [12].Enforcement has been suggested to be important in a number of vertebrate species. One example is found in meerkats, where the dominant female suppresses reproduction in her subordinates [73]. If a subordinate female becomes pregnant when the dominant is also pregnant, then the dominant is likely to subject the subordinate to aggressive attack and temporarily evict her from the group, which usually leads to abortion of the subordinate's litter. Enforcement has also been suggested to be an important selective force for cooperation in humans. In one study, students were split into groups of four to play a public goods game for cash prizes, where each person could contribute monetary units to a group project [74]. The experiment was repeated with and without punishment; punishment was incorporated by allowing individuals to pay money to have units deduced from other players, after they were informed about each other's investments. As expected, punishment led to higher levels of cooperation [74] (Figure 7A).Download high-res image (437KB)Download full-size imageFigure 7. Enforcing cooperation.(A) Humans show higher levels of cooperation in economic games when there are opportunities to punish individuals who do not cooperate. Reproduced with permission from [74]. (B) Lower levels of worker reproduction (cheating) are observed in wasp and bee species where worker policing is more effective [86]. The effectiveness of policing is measured by probability of worker-laid eggs being killed relative to queen-laid eggs. Reproduced with permission from [86]. (C) Legumes sanction rhizobia bacteria that do not fix nitrogen for them [91]. The plant reduces the oxygen supply to nodules where air (N2-O2) is replaced by a gas mixture (Ar-O2) which contains only traces of nitrogen, thus leading to a decrease in rhizobial growth. Reproduced with permission from [91].Enforcement can also explain cooperation between species. An elegant example is provided by the cleaner fish Labroides dimidiatus, which removes and eats ectoparasites from its client reef fish. Although parasite removal and food acquisition are clearly beneficial to the client and cleaner, respectively, there is a conflict, because the cleaners would prefer to eat the tissue or mucus of their hosts, which is costly to the host [75]. The clients use three mechanisms to suppress this conflict and enforce cooperative feeding on ectoparasites only: avoiding cleaners that they have observed cheating (partner choice), leaving for another cleaner (partner switching), and aggressively chasing the cleaner (punishment) 75, 76. Observational and experimental data suggest that cleaner fish are more cooperative and less likely to feed on mucus after punishment 75, 76.Why Enforce?Whilst it is clear that behaviours such as punishment or policing favour cooperation, it is sometimes less obvious why they will be favoured by selection. In order to be favoured, such behaviours must provide a direct or an indirect fitness benefit to the punisher 77, 78. The simplest way in which they could provide a direct fitness advantage is if the behaviours led to the termination of interactions with relatively uncooperative individuals (ostracism) and hence allowed interactions to be focused on more cooperative individuals 57, 65, 79, 80, 81. This mechanism appears to be operating in the cleaner fish discussed above, the legume-rhizobia interaction (see below), the Yucca-Yucca moth interaction [82] and in humans [83]. In meerkats, pregnant subordinates will kill other young — even those of the dominant — and so the dominant increases the survival of her offspring by harassing and evicting pregnant subordinates [73]. A more complicated possibility is that the punished individuals change their behaviour in response to punishment, and are more likely to cooperate with the punisher in future interactions [84]. The relative importance of such punishment remains a major question — it is at work in cleaner fish and could be important in other cooperatively breeding vertebrates or humans.Enforcement could also be favoured if it provides an indirect fitness benefit 77, 78, 85. The simplest way this could occur is by reducing the fitness of individuals who are competing with relatives and hence freeing up resources for relatives. In some ants, bees and wasps, a fraction of the workers lay their own eggs [86]. Other workers frequently do not tolerate such selfish behaviour and selectively cannibalise or ‘police’ eggs laid by workers. This behaviour is selected for because the policing workers can be more related to the sons of the queen than to the sons of the other workers and because cheating workers raising their own sons can reduce the colony's overall productivity [87]. Across species, it has been shown that there are higher levels of worker cooperation at which policing is more common and effective. Specifically, the proportion of workers who lay eggs is negatively correlated with the probability of worker laid eggs being killed [86] (Figure 7B). One way of conceptualising this is that policing reduces the fitness gains of cheating, which is the same as reducing the cost (c) of cooperating in Hamilton's rule.Fixed Enforcement StrategiesThe two previous sections emphasise how cooperation can be enforced by conditional enforcement of behaviour in response to the level of cooperation shown by others. However, cooperation can also be enforced with fixed strategies that limit the opportunity for competition or cheating 8, 57. If opportunities for competition or cheating are limited, individuals can only increase their own success by increasing the success of their group [57]. Consequently, any mechanism that aligns reproductive interests or represses competition within groups will select for higher levels of cooperation. Fair meiosis may be an example of such a mechanism, selected for because it aligns the reproductive interests of genes in a genome [8]. Although selfish genes that increase their own transmission rate can arise and spread, there is selection for them to be suppressed by genes elsewhere in the genome [88]. Other examples of mechanisms that may have evolved to reduce conflict within organisms include separating symbionts into reproductive germ line lineages, and non-reproductive, somatic lineages [89] as well as the uniparental transmission of cytoplasmic genes, such as mitochondrial genes [90].Things Aren't Always So SimpleAlthough we have emphasised how the mechanisms favouring cooperation can be divided up, these mechanisms are not mutually exclusive and there is considerable scope for interactions between them. The examples above have demonstrated how punishment or policing can favour higher levels of cooperation due to direct or indirect fitness benefits. Such mechanisms of enforcement cut across the direct-indirect fitness distinction, because they can alter the relative cost and benefit of cooperating — the b and c of Hamilton's rule [12]. In many cases, both direct and indirect benefits may be involved. For example, if the rhizobia in a root nodule do not provide nitrogen to their leguminous host plant, the plant reduces the growth rate of the bacteria by decreasing oxygen supply [91] (Figure 7C). In this case, each rhizobium cell is selected to fix nitrogen to avoid both itself and its relatives within the nodule being sanctioned. Another complication is that just as competition between relatives reduces kin selection for cooperation, local competition for resources can also reduce the direct fitness benefits of cooperation [92].Origin versus Maintenance of CooperationIt is useful to distinguish between the evolutionary forces favouring the origin and the subsequent elaboration and maintenance of a trait. However, this distinction is rarely made for cooperation. One issue is that the selective force initially responsible for the evolution of cooperation may differ from that driving the observed level of cooperation. In particular, even when there could eventually be a direct fitness benefit to cooperation, it can be hard or impossible for cooperation to spread initially. This is for instance the case with reciprocity [65], punishment 77, 78and group augmentation [56]. It is, therefore, likely that some level of cooperation may initially arise due to factors such as kin selection or shared interests, and that only after this can punishment or sanctions select for higher levels of cooperation [77]. Analogous arguments have been made for cooperatively breeding vertebrates, social insects and rhizobia 58, 81, 86. Another issue is that any mechanism that reduced symbiont diversity would provide a longer term benefit by more closely aligning the reproductive interests of the symbiont with their host [93]. However, this would only be selected for if it provided an immediate benefit. For example, fungus-growing ants in the genus Acromyrmex remove new strains of fungi from their nest, because it leads to a costly incompatibility reaction with their resident fungus strain [94]. In an accompanying review in this issue, Boomsma discusses how mating systems can influence the origin and maintenance of cooperation [95].What We NeedIt is more important to start with what we don't need [96]. First, we do not need to keep reinventing the wheel with more theoretical models that incorrectly claim to provide a new mechanism for the evolution of cooperation 12, 97, 98. This has especially been a problem with models that examine limited dispersal or group structures 99, 100, 101, 102, 103 and which are, therefore, just reinventing kin selection 12, 97, 98. If links with existing theory are not clarified, this misleads and causes confusion. This is illustrated by a recent review which suggests five mechanisms for the evolution of cooperation [104] — three of these were just different ways of modelling the same thing (kin selection) 97, 105, 106, 107, two were different forms of reciprocity, and hence unlikely to be important outside of humans, and most direct fitness benefits for cooperation were ignored. Second, we do not need redefinitions of terms that already have specific and useful meanings. For example the confusion that has been generated by the various redefinitions of ‘altruism’ since Hamilton's [2] original and extremely useful definition [1].Third, we do not need more convoluted theoretical analyses of games such as the Prisoner's Dilemma, snow drift, etc. This approach was extremely useful in the 1970s and 1980s for illustrating that cooperation was a problem and provides a useful framework for conducting experiments with humans. However, games such as the Prisoner's Dilemma and its solution with various forms of reciprocity make a large number of extremely specific and often unrealistic assumptions. We now have much more general, powerful and often simpler methods for analysing the evolution of social behaviours 11, 108, 109, 110. Furthermore, these other methods allow the biology to lead the mathematics [111], rather than contorting real systems into the form of an artificial game 112, 113, and hence facilitate the empirical application and testing of theory. Fourth, we do not need to waste more time on the group selection debate, which was resolved over 20 years ago 10, 114. Group selection is just an alternative way of doing the maths — most workers prefer the kin selection approach because it is usually simpler, more powerful, easier to link with empirical studies and avoids semantic confusion [1].Now, what do we need? First, we need greater integration between theoretical and empirical work [115]. Much theoretical work is aimed at developing very general models that can be difficult to apply to real systems. A greater emphasis is needed on the development of models that can be applied to and tested in specific systems 53, 116. The usefulness of this approach is clearly demonstrated by the fact that the most successful branches of social-evolution theory, such as sex-ratio evolution, are those where theory and empirical work have been highly integrated 11, 22, 47, 117. The need for testable predictions is particularly great for many direct fitness explanations for cooperation. In addition, theory is required to make better use of real data, by providing methods for estimating parameters such as the various components of inclusive fitness [118], and the extent to which competition between relatives reduces selection for cooperation 36, 119.Second, the possible advantages of less traditional study systems need to be exploited. Previous empirical work has focused on animals, and within them, the Hymenoptera and the cooperatively breeding vertebrates. Presumably this is because of the complexities offered by haplodiploid genetics, an excuse for birdwatching or the glamour of working with fluffy mammals. Far less attention is applied to other species such as termites [120], social spiders [60] or aphids [121]. The amazing opportunities offered by bacteria and other microbes have only just been realised, let alone exploited [9]. Furthermore, apart from work on selfish genetic elements and their suppressors [88], far less attention has been paid to the problems of cooperation that occupy lower levels among the major evolutionary transitions, such as the evolution of multicellularity [122].Third, we need greater unification. In some cases, there is surprisingly little interaction between empirical workers and theoreticians who work on different taxa or in different areas. This lack of unification can lead to the semantic and reinvention-of-theory problems discussed above. Inclusive fitness theory [2]provides a relatively unified body of theory on the evolution of cooperation 1, 12, 32, 55, 57, and the major aim for the future should be to show how this links and differentiates explanations for cooperation across various taxa and levels of biological organisation.Fourth, we need to emphasise both the distinction and interplay between mechanistic (proximate) and evolutionary (ultimate or selective value) approaches. It has long been appreciated in the animal behaviour [123] and evolutionary [124]literature that these are complementary and not competing approaches. Indeed, failing to discriminate these approaches can lead to considerable confusion, as illustrated by the recent literature on cooperation in humans [1]. However, this distinction has also led to research on evolutionary questions tending to ignore mechanistic issues. This is a problem when an understanding of mechanism can help explain the pattern and precision of adaptation [125]. For example, the ability with which ants can recognise kin has been shown to depend upon mechanistic constraints imposed by the cuticular hydrocarbon mechanism underlying this behaviour: ants misestimate how many times their queen has mated when her mates share the same cuticular hydrocarbon profile [22].AcknowledgementsWe thank O. Henderson for illustrations; F. Denison, E. Fehr, N. Mehiabadi & T. Wenseleers for providing data or photographs; K. Boomsma, F. Denison, E. Fehr, J. Field, S. Frank, L. Lehmann, A. Ross-Gillespie, A. Russell & T. Wenseleers for discussion; the Royal Society for funding. We apologise for the fact that space constraints have prevented us from including all the examples we would have liked and that were suggested.References1S.A. West, A.S. Griffin, A. GardnerSocial semantics: altruism, cooperation, mutualism, strong reciprocity and group selectionJ. Evol. Biol., 20 (2007), pp. 415-432CrossRefView Record in ScopusGoogle Scholar2W.D. HamiltonThe genetical evolution of social behaviour, I & IIJ. Theor. Biol., 7 (1964), pp. 1-52View Record in ScopusGoogle Scholar3A.S. Griffin, J.M. Pemberton, P.N.M. Brotherton, G. McIlrath, D. Gaynor, R. Kansky, J.O'Riain, T.H. Clutton-BrockA genetic analysis of breeding success in the cooperative meerkat (Suricata suricatta)Behav. Ecol., 14 (2003), pp. 472-480CrossRefView Record in ScopusGoogle Scholar4G. HardinThe tragedy of the commonsScience, 162 (1968), pp. 1243-1248View Record in ScopusGoogle Scholar5W.D. HamiltonAltruism and related phenomena, mainly in social insectsAnnu. Rev. Ecol. Syst., 3 (1972), pp. 193-232CrossRefView Record in ScopusGoogle Scholar6E.G. LeighGenes, bees and ecosystems: the evolution of a common interest among individualsTrends Ecol. Evol., 6 (1991), pp. 257-262ArticleDownload PDFView Record in ScopusGoogle Scholar7J. Maynard Smith, E. SzathmaryThe Major Transitions in EvolutionW.H. Freeman, Oxford (1995)Google Scholar8E.G. LeighAdaptation and DiversityFreeman, Cooper and Company, San Francisco (1971)Google Scholar9S.A. West, A.S. Griffin, A. Gardner, S.P. DiggleSocial evolution theory for microbesNat. Rev. Microbiol., 4 (2006), pp. 597-607CrossRefView Record in ScopusGoogle Scholar10A. GrafenNatural selection, kin selection and group selectionJ.R. Krebs, N.B. Davies (Eds.), Behavioural Ecology: An Evolutionary Approach (Second Edition), Blackwell Scientific Publications, Oxford, UK (1984), pp. 62-84View Record in ScopusGoogle Scholar11S.A. FrankFoundations of Social EvolutionPrinceton University Press, Princeton, New Jersey (1998)Google Scholar12L. Lehmann, L. KellerThe evolution of cooperation and altruism. A general framework and classification of modelsJ. Evol. Biol., 19 (2006), pp. 1365-1378CrossRefView Record in ScopusGoogle Scholar13J. Maynard SmithGroup selection and kin selectionNature, 201 (1964), pp. 1145-1147Google Scholar14A.F. Russell, B.J. HatchwellExperimental evidence for kin-biased helping in a cooperatively breeding vertebrateProc. Roy. Soc. Lond. B, 268 (2001), pp. 2169-2174CrossRefView Record in ScopusGoogle Scholar15S.P. Sharp, A. McGowan, M.J. Wood, B.J. HatchwellLearned kin recognition cues in a social birdNature, 434 (2005), pp. 1127-1130CrossRefView Record in ScopusGoogle Scholar16A.S. Griffin, S.A. WestKin discrimination and the benefit of helping in cooperatively breeding vertebratesScience, 302 (2003), pp. 634-636CrossRefView Record in ScopusGoogle Scholar17T.H. Clutton-Brock, P.N.M. Brotherton, M.J. Oriain, A.S. Griffin, D. Gaynor, L. Sharpe, R. Kansky, M.B. Manser, G.M. McIlrathIndividual contributions to babysitting in a cooperative mongoose, Suricata suricattaProc. R. Soc. Lond. B, 267 (2000), pp. 301-305CrossRefView Record in ScopusGoogle Scholar18N.J. Mehdiabadi, C.N. Jack, T.T. Farnham, T.G. Platt, S.E. Kalla, G. Shaulsky, D.C.Queller, J.S. StrassmanKin preference in a social microbeNature, 442 (2006), pp. 881-882CrossRefView Record in ScopusGoogle Scholar19A. GrafenDo animals really recognise kin?Anim. Behav., 39 (1990), pp. 42-54ArticleDownload PDFView Record in ScopusGoogle Scholar20H. Helanterä, L. SundströmWorker policing and nest mate recognition in the ant Formica fuscaBehav. Ecol. Sociobiol., 61 (2007), pp. 1143-1149CrossRefView Record in ScopusGoogle Scholar21D. Lieberman, J. Tooby, L. CosmidesDoes morality have a biological basis? An empirical test of the factors governing moral sentiments relating to incestProc. Roy. Soc. Lond. B, 270 (2003), pp. 819-826CrossRefView Record in ScopusGoogle Scholar22J.J. Boomsma, J. Nielsen, L. Sundstrom, N.J. Oldham, J. Tentschert, H.C. Petersen, E.D.MorganInformational constraints on optimal sex allocation in antsProc. Natl. Acad. Sci. USA, 100 (2003), pp. 8799-8804CrossRefView Record in ScopusGoogle Scholar23J.M. MateoKin-recognition abilities and nepotism as a function of socialityProc. Roy. Soc. Lond. B, 269 (2002), pp. 721-727CrossRefView Record in ScopusGoogle Scholar24Rousset, F., and Roze, D., (2007). Constraints on the origin and maintenance of genetic kin recognition. Evolution, Submitted.Google Scholar25L. KellerIndiscriminate altruism: unduly nice parents and siblingsTrends Ecol. Evol., 12 (1997), pp. 99-103ArticleDownload PDFView Record in ScopusGoogle Scholar26J.S. Strassman, C.J. Klingler, E. Arévalo, F. Zacchi, A. Husain, J. Williams, P. Seppä, D.C.QuellerAbsence of within-colony kin discrimination in behavioural interactions of swarm-founding waspsProc. Roy. Soc. Lond. B, 264 (1997), pp. 1565-1570Google Scholar27S.E. Reece, D.M. Shuker, I. Pen, A.B. Duncan, A. Choudhary, C.M. Batchelor, S.A. WestKin discrimination and sex ratios in a parasitoid waspJ. Evol. Biol., 17 (2004), pp. 208-216View Record in ScopusGoogle Scholar28B. Holzer, R. Kümmerli, L. Keller, M. ChapuisatSham nepotism as a result of intrinsic differences in brood viability in antsProc. Roy. Soc. Lond. B, 273 (2006), pp. 2049-2052CrossRefView Record in ScopusGoogle Scholar29R. Axelrod, R.A. Hammond, A. GrafenAltruism via kin-selection strategies that rely on arbitrary tags with which they coevolveEvolution, 58 (2004), pp. 1833-1838CrossRefView Record in ScopusGoogle Scholar30W.D. HamiltonInnate social aptitudes of man: An approach from evolutionary geneticsR. Fox (Ed.), Biosocial Anthropology, Wiley, New York, NY (1975), pp. 133-155View Record in ScopusGoogle Scholar31R. DawkinsThe Selfish GeneOxford University Press, Oxford, UK (1976)Google Scholar32A. GrafenOptimisation of inclusive fitnessJ. Theoret. Biol., 238 (2006), pp. 541-563ArticleDownload PDFView Record in ScopusGoogle Scholar33H. Helanterä, K. BargumPedigree relatedness, not greenbeard genes, explains eusocialityOikos, 116 (2007), pp. 217-220CrossRefView Record in ScopusGoogle Scholar34D.C. Queller, E. Ponte, S. Bozzaro, J.E. StrassmannSingle-gene greenbeard effects in the social amoeba Dictostelium discoideumScience, 299 (2003), pp. 105-106CrossRefView Record in ScopusGoogle Scholar35A.S. Griffin, S.A. West, A. BucklingCooperation and competition in pathogenic bacteriaNature, 430 (2004), pp. 1024-1027CrossRefView Record in ScopusGoogle Scholar36S.A. West, I. Pen, A.S. GriffinCooperation and competition between relativesScience, 296 (2002), pp. 72-75CrossRefView Record in ScopusGoogle Scholar37W.D. HamiltonSelection of selfish and altruistic behaviour in some extreme modelsJ.F. Eisenberg, W.S. Dillon (Eds.), Man and Beast: Comparative Social Behavior, Smithsonian Press, Washington, DC (1971), pp. 57-91View Record in ScopusGoogle Scholar38S.A. West, M.G. Murray, C.A. Machado, A.S. Griffin, E.A. HerreTesting Hamilton's rule with competition between relativesNature, 409 (2001), pp. 510-513CrossRefView Record in ScopusGoogle Scholar39P.D. TaylorAltruism in viscous populations - an inclusive fitness modelEvol. Ecol., 6 (1992), pp. 352-356CrossRefView Record in ScopusGoogle Scholar40A. Gardner, S.A. WestDemography, altruism, and the benefits of buddingJ. Evol. Biol., 19 (2006), pp. 1707-1716CrossRefView Record in ScopusGoogle Scholar41L. Lehmann, N. Perrin, F. RoussetPopulation demography and the evolution of helping behavioursEvolution, 60 (2006), pp. 1137-1151CrossRefView Record in ScopusGoogle Scholar42A. Gardner, S.A. WestSpite and the scale of competitionJ. Evol. Biol., 17 (2004), pp. 1195-1203CrossRefView Record in ScopusGoogle Scholar43D. Giron, D.W. Dunn, I.C.W. Hardy, M.R. StrandAggression by polyembryonic wasp soldiers correlates with kinship but not resource competitionNature, 430 (2004), pp. 676-679CrossRefView Record in ScopusGoogle Scholar44A. Gardner, I.C.W. Hardy, P.D. Taylor, S.A. WestSpiteful soldiers and sex ratio conflict in polyembryonic parasitoid waspsAm. Nat., 169 (2007), pp. 519-533CrossRefView Record in ScopusGoogle Scholar45M.J. West-EberhardThe evolution of social behavior by kin selectionQ. Rev. Biol., 50 (1975), pp. 1-33View Record in ScopusGoogle Scholar46A.S. Griffin, S.A. WestKin selection: Fact and fictionTrends Ecol. Evol., 17 (2002), pp. 15-21ArticleDownload PDFView Record in ScopusGoogle Scholar47R.L. Trivers, H. HareHaplodiploidy and the evolution of the social insectsScience, 191 (1976), pp. 249-263View Record in ScopusGoogle Scholar48J.W. Stubblefield, E.L. CharnovSome conceptual issues in the origin of eusocialityHeredity, 57 (1986), pp. 181-187CrossRefView Record in ScopusGoogle Scholar49D.C. Queller, J.E. StrassmannKin selection and social insectsBiocscience, 48 (1998), pp. 165-175CrossRefView Record in ScopusGoogle Scholar50E.O. Wilson, B. HölldoblerEusociality: origin and consequencesProc. Natl. Acad. Sci. USA, 102 (2005), pp. 13367-13371CrossRefView Record in ScopusGoogle Scholar51K.R. Foster, T. Wenseleers, F.L.W. RatnieksKin selection is the key to altruismTrends Ecol. Evol., 21 (2006), pp. 57-60ArticleDownload PDFView Record in ScopusGoogle Scholar52J. Field, A. Cronin, C. BridgeFuture fitness and helping in social queuesNature, 441 (2006), pp. 214-217CrossRefView Record in ScopusGoogle Scholar53M.A. Cant, J. FieldHelping effort and future fitness in cooperative animal societiesProc. Roy. Soc. Lond. B, 268 (2001), pp. 1959-1964CrossRefView Record in ScopusGoogle Scholar54R.L. TriversThe evolution of reciprocal altruismQ. Rev. Biol., 46 (1971), pp. 35-57CrossRefGoogle Scholar55J.L. Sachs, U.G. Mueller, T.P. Wilcox, J.J. BullThe evolution of cooperationQ. Rev. Biol., 79 (2004), pp. 135-160CrossRefView Record in ScopusGoogle Scholar56H. Kokko, R.A. Johnstone, T.H. Clutton-BrockThe evolution of cooperative breeding through group augmentationProc. R. Soc. Lond. B, 268 (2001), pp. 187-196CrossRefView Record in ScopusGoogle Scholar57S.A. FrankRepression of competition and the evolution of cooperationEvolution, 57 (2003), pp. 693-705CrossRefView Record in ScopusGoogle Scholar58T.H. Clutton-BrockBreeding together: kin selection, reciprocity and mutualism in cooperative animal societiesScience, 296 (2002), pp. 69-72CrossRefView Record in ScopusGoogle Scholar59T.H. Clutton-Brock, A.F. Russell, L.L. Sharpe, A.J. Young, Z. Balmforth, G.M. McllrathEvolution and development of sex differences in cooperative behavior in MeerkatsScience, 297 (2002), pp. 253-256CrossRefView Record in ScopusGoogle Scholar60L. Avilés, P. TufiñoColony size and individual fitness in the social spider Anelosimus eximiusAm. Nat., 152 (1998), pp. 403-418CrossRefView Record in ScopusGoogle Scholar61G. Bernasconi, J.E. StrassmanCooperation among unrelated individuals: the ant foundress caseTrends Ecol. Evol., 14 (1999), pp. 477-482ArticleDownload PDFView Record in ScopusGoogle Scholar62K.R. Foster, T. WenseleersA general model for the evolution of mutualismsJ. Evol. Biol., 19 (2006), pp. 1283-1293CrossRefView Record in ScopusGoogle Scholar63D.C. Queller, F. Zacchi, R. Cervo, S. Turillazzi, M.T. Henshaw, L.A. Santorelli, J.E.StrassmannUnrelated helpers in a social insectNature, 405 (2000), pp. 784-787CrossRefView Record in ScopusGoogle Scholar64F.R. Dani, K.R. Foster, F. Zacchi, P. Seppä, A. Massolo, A. Carelli, E. Arévalo, D.C. Queller, J.E. Strassman, S. TurillazziCan cuticular lipids provide sufficient information for within-colony nepotism in wasps?Proc. Roy. Soc. Lond. B, 271 (2004), pp. 745-753CrossRefView Record in ScopusGoogle Scholar65R. Axelrod, W.D. HamiltonThe evolution of cooperationScience, 211 (1981), pp. 1390-1396View Record in ScopusGoogle Scholar66R.D. AlexanderThe evolution of social behaviorAnnu. Rev. Ecol. Syst., 5 (1974), pp. 325-383CrossRefView Record in ScopusGoogle Scholar67R.D. AlexanderThe Biology of Moral SystemsAldine de Gruyter, New York (1987)Google Scholar68M.A. Nowak, K. SigmundEvolution of indirect reciprocity by image scoringNature, 393 (1998), pp. 573-577CrossRefView Record in ScopusGoogle Scholar69L.A. DugatkinCooperation Among Animals: An Evolutionary PerspectiveOxford University Press, Oxford, UK (1997)Google Scholar70P. HammersteinGenetic and Cultural Evolution of CooperationMIT Press, Cambridge, UK (2003)Google Scholar71J.R. Stevens, M.D. HauserWhy be nice? Psychological constraints on the evolution of cooperationTrends Cognitive Sci., 8 (2004), pp. 60-65ArticleDownload PDFView Record in ScopusGoogle Scholar72R. Bergmüller, R. Bshary, R.A. Johnstone, A.F. RussellIntegrating coopeative breeding and cooperation theoryBehav. Proc. (2007)In pressGoogle Scholar73A.J. Young, A.A. Carlson, S.L. Monfort, A.F. Russell, N.C. Bennett, T.H. Clutton-BrockStress and the suppression of subordinate reproduction in cooperatively breeding meerkatsProc. Natl. Acad. Sci. USA, 103 (2006), pp. 12005-12010CrossRefView Record in ScopusGoogle Scholar74E. Fehr, S. GächterAltruistic punishment in humansNature, 415 (2002), pp. 137-140CrossRefView Record in ScopusGoogle Scholar75R. Bshary, A.S. GrutterAsymetric cheating opportunities and partner control in a cleaner fish mutualismAnimal Behaviour, 63 (2002), pp. 547-555ArticleDownload PDFView Record in ScopusGoogle Scholar76R. Bshary, A.S. GrutterPunishment and partner switching cause cooperative behaviour in a cleaning mutualismBiol. Letters, 1 (2005), pp. 396-399CrossRefView Record in ScopusGoogle Scholar77A. Gardner, S.A. WestCooperation and punishment, especially in humansAm. Nat., 164 (2004), pp. 753-764CrossRefView Record in ScopusGoogle Scholar78L. Lehmann, F. Rousset, D. Roze, L. KellerStrong-reciprocity or strong-ferocity? A population genetic view of the evolution of altruistic punishmentAm. Nat. (2007)in pressGoogle Scholar79M.G. MurrayFigs (Ficus spp.) and fig wasps (Chalcidoidea, Agaonidae): hypotheses for an ancient symbiosisBiol. J. Linnean Soc., 26 (1985), pp. 69-81CrossRefView Record in ScopusGoogle Scholar80J.J. Bull, W.R. RiceDistinguishing mechanisms for the evolution of cooperationJ. Theor. Biol., 149 (1991), pp. 63-74ArticleDownload PDFView Record in ScopusGoogle Scholar81S.A. West, E.T. Kiers, I. Pen, R.F. DenisonSanctions and mutualism stability: when should less beneficial mutualists be tolerated?J. Evol. Biol., 15 (2002), pp. 830-837CrossRefView Record in ScopusGoogle Scholar82O. Pellmyr, C.J. HuthEvolutionary stability of mutualism between yuccas and yucca mothsNature, 372 (1994), pp. 257-260CrossRefView Record in ScopusGoogle Scholar83M.J.F. Brown, A. Falk, E. FehrRelational contracts and the nature of market interactionsEcon., 72 (2004), pp. 747-780View Record in ScopusGoogle Scholar84T.H. Clutton-Brock, G.A. ParkerPunishment in animal societiesNature, 373 (1995), pp. 209-216CrossRefView Record in ScopusGoogle Scholar85F.L.W. RatnieksReproductive harmony via mutual policing by workers in eusocial HymenopteraAm. Nat., 132 (1988), pp. 217-236View Record in ScopusGoogle Scholar86T. Wenseleers, F.L.W. RatnieksEnforced altruism in insect societiesNature, 444 (2006), p. 50CrossRefView Record in ScopusGoogle Scholar87T. Wenseleers, H. Helantera, A. Hart, F.L.W. RatnieksWorker reproduction and policing in insect societies: An ESS analysisJ. Evol. Biol., 17 (2004), pp. 1035-1047CrossRefView Record in ScopusGoogle Scholar88A. Burt, R. TriversGenes in Conflict: The Biology of Selfish Genetic ElementsHarvard University Press, Cambridge, Massachusetts (2006)Google Scholar89S.A. FrankHost control of symbiont transmission: the separation of symbionts into germ and somaAm. Nat., 148 (1996), pp. 1113-1124CrossRefView Record in ScopusGoogle Scholar90L.D. Hurst, W.D. HamiltonCytoplasmic fusion and the nature of the sexesProc. Roy. Soc. Lond. B, 247 (1992), pp. 189-194View Record in ScopusGoogle Scholar91E.T. Kiers, R.A. Rousseau, S.A. West, R.F. DenisonHost sanctions and the legume-rhizobium mutualismNature, 425 (2003), pp. 78-81CrossRefView Record in ScopusGoogle Scholar92S.A. West, A. Gardner, D.M. Shuker, T. Reynolds, M. Burton-Chellow, E.M. Sykes, M.A.Guinnee, A.S. GriffinCooperation and the scale of competition in humansCurr. Biol., 16 (2006), pp. 1103-1106ArticleDownload PDFView Record in ScopusGoogle Scholar93S.A. FrankHost-symbiont conflict over the mixing of symbiotic lineagesProc. Roy. Soc. Lond. B, 263 (1996), pp. 339-344View Record in ScopusGoogle Scholar94M. Poulsen, J.J. BoomsmaMutualistic fungi control crop diversity in fungus growing antsScience, 307 (2005), pp. 741-744CrossRefView Record in ScopusGoogle Scholar95J.J. BoomsmaKin selection versus sexual selection: why the ends do not meetCurr. Biol., 17 (2007), pp. R673-R683ArticleDownload PDFView Record in ScopusGoogle Scholar96A.S. KondrashovClassification of hypotheses on the advantage of amphimixisJ. Heredity, 84 (1993), pp. 372-387CrossRefView Record in ScopusGoogle Scholar97L. Lehmann, L. Keller, S.A. West, D. RozeGroup selection and kin selection. Two concepts but one processProc. Natl. Acad. Sci. USA, 104 (2007), pp. 6736-6739CrossRefView Record in ScopusGoogle Scholar98A. GrafenDetecting kin selection at work using inclusive fitnessProc. Roy. Soc. Lond. B, 274 (2007), pp. 713-719CrossRefView Record in ScopusGoogle Scholar99M.A. Nowak, R.M. MayEvolutionary games and spatial chaosNature, 359 (1992), pp. 826-829CrossRefView Record in ScopusGoogle Scholar100M.A. Nowak, S. Bonhoeffer, R.M. MaySpatial games and the maintenance of cooperationProc. Natl. Acad. Sci. USA, 91 (1994), pp. 4877-4881CrossRefView Record in ScopusGoogle Scholar101E. Lieberman, C. Hauert, M.A. NowakEvolutionary dynamics on graphsNature, 433 (2005), pp. 312-316CrossRefView Record in ScopusGoogle Scholar102T. Killingback, J. Bieri, T. FlattEvolution in group-structured populations can resolve the tragedy of the commonsProc. Roy. Soc. Lond. B, 273 (2006), pp. 1477-1481CrossRefView Record in ScopusGoogle Scholar103A. Traulsen, M.A. NowakEvolution of cooperation by multilevel selectionProc. Natl. Acad. Sci. USA, 103 (2006), pp. 10952-10955CrossRefView Record in ScopusGoogle Scholar104M.A. NowakFive rules for the evolution of cooperationScience, 314 (2006), pp. 1560-1563CrossRefView Record in ScopusGoogle Scholar105A. GrafenAn inclusive fitness analysis of altruism on a cyclical networkJ. Evol. Biol. (2007)In pressGoogle Scholar106L. Lehmann, L. Keller, D.J.T. SumpterInclusive fitness explains the evolution of helping and harming behaviors on graphsJ. Evol. Biol. (2007)In pressGoogle Scholar107P.D. Taylor, T. Day, G. WildEvolution of cooperation in a finite homogeneous graphNature, 447 (2007), pp. 469-472CrossRefView Record in ScopusGoogle Scholar108P.D. TaylorInclusive fitness arguments in genetic models of behaviourJ. Math. Biol., 34 (1996), pp. 654-674CrossRefView Record in ScopusGoogle Scholar109F. RoussetGenetic Structure and Selection in Subdivided PopulationsPrinceton University Press, Princeton, NJ (2004)Google Scholar110A. Gardner, S.A. West, N.H. BartonThe relation between multilocus population genetics and social evolution theoryAm. Nat., 169 (2007), pp. 207-226CrossRefView Record in ScopusGoogle Scholar111S.P. BrownCollective action in an RNA virusJ. Evol. Biol., 14 (2001), pp. 821-828View Record in ScopusGoogle Scholar112P.E. Turner, L. ChaoPrisoner's dilemma in an RNA virusNature, 398 (1999), pp. 441-443CrossRefView Record in ScopusGoogle Scholar113M. Doebeli, C. HauertModels of cooepration based on the Prisoner's dilemma and snowdrift gamesEcol. Letters, 8 (2005), pp. 748-766CrossRefView Record in ScopusGoogle Scholar114S.A. FrankHierarchical selection theory and sex ratios. I. General solutions for structured populationsTheoret. Popul. Biol., 29 (1986), pp. 312-342ArticleDownload PDFView Record in ScopusGoogle Scholar115O. Leimar, P. HammersteinFacing the factsJ. Evol. Biol., 19 (2006), pp. 1403-1405CrossRefView Record in ScopusGoogle Scholar116A. Ross-Gillespie, A. Gardner, S.A. West, A.S. GriffinFrequency dependence and cooperation: theory and a test with bacteriaAm. Nat. (2007)In pressGoogle Scholar117S.A. West, D.M. Shuker, B.C. SheldonSex ratio adjustment when relatives interact: a test of constraints on adaptationEvolution, 59 (2005), pp. 1211-1228CrossRefView Record in ScopusGoogle Scholar118M.K. OliHamilton goes empirical: estimation of inclusive fitness from life-history dataProc. Roy. Soc. Lond. B, 270 (2003), pp. 307-311CrossRefView Record in ScopusGoogle Scholar119D.C. QuellerGenetic relatedness in viscous populationsEvol. Ecol., 8 (1994), pp. 70-73CrossRefView Record in ScopusGoogle Scholar120B.L. ThorneEvolution of eusociality in termitesAnnu. Rev. Ecol. Syst., 28 (1997), pp. 27-54CrossRefView Record in ScopusGoogle Scholar121D.L. Stern, W.A. FosterThe evolution of soldiers in aphidsBiol. Rev., 71 (1996), pp. 27-79CrossRefView Record in ScopusGoogle Scholar122R.E. Michod, D. RozeCooperation and conflict in the evolution of multicellularityHeredity, 86 (2001), pp. 1-7CrossRefView Record in ScopusGoogle Scholar123N. TinbergenOn aims and methods of ethologyZeitschrift fur Tierpsychologie, 20 (1963), pp. 410-433View Record in ScopusGoogle Scholar124E. MayrCause and effect in biologyScience, 134 (1961), pp. 1501-1506View Record in ScopusGoogle Scholar125D.M. Shuker, S.A. WestInformation constraints and the precision of adaptation: sex ratio manipulation in waspsProc. Natl. Acad. Sci. USA, 101 (2004), pp. 10363-10367Source: Evolutionary Explanations for Cooperation
How do you figure a profit using a quadratic equation?
Profit is the difference between the amount of money made, called revenue, and the costs involved in making that money. So, if we let profit be labeled [math]y[/math], then:[math]y=R-C[/math]where [math]R[/math] is revenue and [math]C[/math] is cost.To make money, let’s assume that our business sells cellphones. The quantity of cellphones that customers will buy depends on the price. If the cellphone is very expensive, then not many people can afford it, so we sell only a small number of cellphones at the high price, and our revenue is that price, [math]p[/math], times the number of cellphones sold, [math]x[/math], for a revenue of [math]px[/math]. But, if we reduce our price, we can sell more cellphones. After some research, we discover that we can model the price we can get, as a function of the number of cellphones sold, as a line that slopes downward and the equation for the line is:[math]p=a-bx[/math]We notice that when the cellphone price [math]p[/math] is high at [math]a[/math], we sell no cellphones because nobody is willing to pay that much, and when [math]p=0[/math] (i.e., we give them away), that the quantity we give away is [math]\frac{a}{b}[/math] due to the limited size of our market. So, we can write our revenue as:[math]R=px=(a-bx)x=ax-bx^2[/math]It costs money to make cellphones. There are two types of costs. First, we need to set up our factory, design the cellphone, and the create the process to make the cellphone, and to maintain this infrastructure for every year that we are in business. This is a large yearly fixed cost that will not change relative to the number of cellphones that we make and we need to spend this money before we can make a single cell. Let’s call this fixed cost [math]f[/math]. Then, for each cellphone that we make, we will need to buy materials from which it will be made and pay workers who will make it. These costs vary depending on the number of cellphones that we make, and are called variable costs. If the cost to make a cellphone is [math]v[/math], then the variable cost to make [math]x[/math] cellphones is [math]vx[/math], and the total cost will be the sum of the fixed cost to set up and maintain our factory and the variable cost to make the number of cellphones that we decide to make:[math]C=f+vx[/math]We can now find the equation for profit by substituting these relationships into the equation for profit:[math]y=R-C=(ax-bx^2)-(f+vx)[/math]and simplifying, we get:[math]y=-bx^2+(a-v)x-f[/math]which is a quadratic equation. Since the [math]x^2[/math]-term is negative, we see that this equation is a downward opening parabola. The profit, [math]y[/math], will be maximum at the vertex of this parabola. If we put this equation into the vertex form for a parabola, we can see the quantity, [math]x_{max}[/math], that we need to sell for the maximum profit, and what that maximum profit will be. To do this, without using calculus, we complete the square:[math]y=-b(x^2-\frac{(a-v)}{b}+[-\frac{a-v}{2b}]^2-[-\frac{a-v}{2b}]^2)-f[/math][math]y=-b(x^2-\frac{(a-v)}{b}+[-\frac{a-v}{2b}]^2)+b[-\frac{a-v}{2b}]^2)-f[/math][math]y=-b(x-\frac{(a-v)}{2b})^2+\frac{(a-v)^2}{4b}-f[/math]From this form, we see that the profit y is maximum when the squared term is zero because that term is negative and acts to reduce profit. From this fact, we can determine the quantity that we need to sell to maximize our profit by setting the squared term equal to zero and solving for [math]x[/math]:[math]x_{max}-\frac{(a-v)}{2b}=0[/math][math]x_{max}=\frac{(a-v)}{2b}[/math]and that our maximum profit will then be:[math]y_{max}=\frac{(a-v)^2}{4b}-f[/math]From our discussion above, [math]a[/math] and [math]b[/math] depend on the market and our ability to reach customers, while [math]v[/math] and [math]f[/math] depend on our ability to control costs.Suppose that nobody will pay [math]$3000[/math] for a cellphone and that, at most, we could give away [math]10,000,000[/math] free cellphones in our market each year. Then, [math]a=3000[/math] and [math]b=0.0003[/math] and our revenue would be:[math]R=3000x-0.0003x^2[/math]Further, suppose that it will cost us [math]$500,000,000[/math] to build and maintain a factory with all of it overhead for each year, and it will [math]$150[/math] to make each cellphone. Then, our costs would be:[math]C=500000000+150x[/math]From this, we see that we need to produce:[math]x_{max}=\frac{3000–150}{2(0.0003)}=4,750,000[/math] cellphonesand sell them for:[math]p=3000–0.0003(4750000)=$1575[/math] eachto maximize our profit of:[math]y_{max}=\frac{(3000–150)^2}{4(0.0003)}-500000000=$6,268,750,000[/math]Our yearly revenue will be:[math]R=$7,481,250,000[/math]and our yearly costs will be:[math]C=$1,212,500,000[/math]We can also determine the minimum number of cellphones that we need to sell to make any profit at all. This is called the breakeven quantity and is found from the roots of our parabola equation:[math]y=-bx^2+(a-v)x-f=0[/math]which are:[math]x_{be}=\frac{(v-a)\pm\sqrt{(a-v)^2–4(-b)(-f)}}{-2b}[/math]We will need to sell at least [math]178,804[/math] cellphones at [math]$2946.36[/math], or at least [math]9,321,196[/math] cellphones at [math]$203.64[/math] each, to cover our costs.
- Home >
- Catalog >
- Miscellaneous >
- Manual Sample >
- Technical Manual Sample >
- Q-see Technical Manual Sample >
- Form 4b Factory Act