How to Edit The 2 Chromosomes And Mitosis Answer Key and make a signature Online
Start on editing, signing and sharing your 2 Chromosomes And Mitosis Answer Key online following these easy steps:
- Push the Get Form or Get Form Now button on the current page to jump to the PDF editor.
- Wait for a moment before the 2 Chromosomes And Mitosis Answer Key is loaded
- Use the tools in the top toolbar to edit the file, and the edits will be saved automatically
- Download your completed file.
The best-rated Tool to Edit and Sign the 2 Chromosomes And Mitosis Answer Key
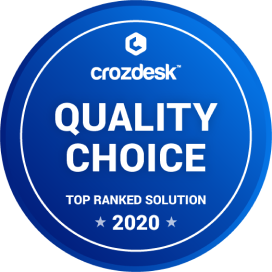
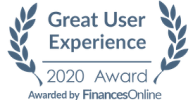
A quick tutorial on editing 2 Chromosomes And Mitosis Answer Key Online
It has become quite easy presently to edit your PDF files online, and CocoDoc is the best free app you have ever seen to make a lot of changes to your file and save it. Follow our simple tutorial to start!
- Click the Get Form or Get Form Now button on the current page to start modifying your PDF
- Add, change or delete your text using the editing tools on the toolbar above.
- Affter altering your content, put on the date and create a signature to bring it to a perfect comletion.
- Go over it agian your form before you click on the button to download it
How to add a signature on your 2 Chromosomes And Mitosis Answer Key
Though most people are adapted to signing paper documents using a pen, electronic signatures are becoming more usual, follow these steps to add an online signature!
- Click the Get Form or Get Form Now button to begin editing on 2 Chromosomes And Mitosis Answer Key in CocoDoc PDF editor.
- Click on the Sign tool in the toolbar on the top
- A window will pop up, click Add new signature button and you'll have three ways—Type, Draw, and Upload. Once you're done, click the Save button.
- Drag, resize and settle the signature inside your PDF file
How to add a textbox on your 2 Chromosomes And Mitosis Answer Key
If you have the need to add a text box on your PDF so you can customize your special content, do the following steps to get it done.
- Open the PDF file in CocoDoc PDF editor.
- Click Text Box on the top toolbar and move your mouse to position it wherever you want to put it.
- Write in the text you need to insert. After you’ve typed in the text, you can select it and click on the text editing tools to resize, color or bold the text.
- When you're done, click OK to save it. If you’re not happy with the text, click on the trash can icon to delete it and start afresh.
A quick guide to Edit Your 2 Chromosomes And Mitosis Answer Key on G Suite
If you are looking about for a solution for PDF editing on G suite, CocoDoc PDF editor is a recommendable tool that can be used directly from Google Drive to create or edit files.
- Find CocoDoc PDF editor and install the add-on for google drive.
- Right-click on a PDF document in your Google Drive and select Open With.
- Select CocoDoc PDF on the popup list to open your file with and allow CocoDoc to access your google account.
- Modify PDF documents, adding text, images, editing existing text, mark up in highlight, erase, or blackout texts in CocoDoc PDF editor before saving and downloading it.
PDF Editor FAQ
What are the differences between mitosis and meiosis?
Answer for Class 11 Biology StudentsHere I am mentioning general differences and also comparing phases of mitosis and meiosis.Mitosis1. Takes place in somatic cells.2. Cells undergoing mitosis may be 2n or n.3. Mitosis is comparatively simple and takes less time to complete.4. Number of chromosomes remains the same after mitosis.5. Produces no variations.6. Mitosis takes part in healing and repairing.Prophase:1. It is simple and can not be distinguished into sub stages.2. Pairing of chromosomes does not occur in mitosis.3. Synaptinemal complex is absent.4. Recombination is absent.5. Chiasmata are absent.Metaphase:1. Centromeres produce single metaphasic plate.2. A centromere is connected with both spindle poles.3. Two chromatids of chromosomes are genetically similar.Anaphase:1. Centromere splits into two in the beginning of the anaphase.2. Anaphasic chromosomes are single stranded.3. Similar chromosomes move towards opposite poles in anaphase.Meiosis1. Occurs in reproductive cells.2. Cells undergoing meiosis are always 2n3. Meiosis is complicated and takes longer time to complete.4. Number of chromosomes reduces to halfafter meiosis.5. It introduces variations.6. Meiosis takes part in formation of gametes and maintenance of chromosome number of species.Prophase1. Prophase I is complicated and is divided into 5 sub stages.2. Synapsis of homologous chromosomes takes place during zygotene.3. Synapsed homologous chromosomes develop synaptinemal complex.4. Recombination occurs between non sister chromatids of homologous chromosomes during pachytene stage.5. Chiasmata are visible during diplotene stage.Metaphase1. Double metaphasic plate is formed during metaphase I but one plate during metaphase II.2. A centromere is connected to one spindle pole in metaphase I but both in metaphase II.3. Two chromatids of a chromosome are genetically dissimilar due to recombination.Anaphase1. Centromeres do not divide during anaphase I but do so in anaphase II.2. Chromosomes are double stranded in anaphase I but single stranded in anaphase II.3. Dissimilar chromosomes move towards opposite poles.If you find the answer useful, plz upvote it.
How many centromeres are present in a cell at anaphase I?
Each of us has enough DNA to reach from here to the sun and back, more than 300 times. How is all of that DNA packaged so tightly into chromosomes and squeezed into a tiny nucleus?The answer to this question lies in the fact that certain proteins compact chromosomal DNA into the microscopic space of the eukaryotic nucleus. These proteins are called histones, and the resulting DNA-protein complex is called chromatin. It may seem paradoxical that proteins are added to DNA to make it more compact. However, if you have ever tried to store a garden hose, you know that it is much easier to do so if you begin by coiling the hose. Of course, coiling requires work, and energy is needed to perform work. Thus, within the nucleus, histones provide the energy (mainly in the form of electrostatic interactions) to fold DNA. As a result, chromatin can be packaged into a much smaller volume than DNA alone.Histones are a family of small, positively charged proteins termed H1, H2A, H2B, H3, and H4 (Van Holde, 1988). DNA is negatively charged, due to the phosphate groups in its phosphate-sugar backbone, so histones bind with DNA very tightly.Chromosomes Are Most Compacted During MetaphaseWhen eukaryotic cells divide, genomic DNA must be equally partitioned into both daughter cells. To accomplish this, the DNA becomes highly compacted into the classic metaphase chromosomes that can be seen with a light microscope. Once a cell has divided, its chromosomes uncoil again.Comparing the length of metaphase chromosomes to that of naked DNA, the packing ratio of DNA in metaphase chromosomes is approximately 10,000:1 (depending on the chromosome). This can be thought of as akin to taking a rope as long as a football field and compacting it down to less than half an inch. This level of compaction is achieved by repeatedly folding chromatin fibres into a hierarchy of multiple loops and coils (Figure 1). Exactly how this is accomplished is unclear, but the phosphorylation of histone H1 may play a role. Indeed, this is just one area of DNA packaging that researchers will continue to explore in the years to come.[By Anthony T. Annunziato, Ph.D. (Biology Department, Boston College) © 2008 Nature Education Citation: Annunziato, A. (2008) DNA Packaging: Nucleosomes and Chromatin. Nature Education 1(1):26]Source of the above information and images:DNA Packaging: Nucleosomes and ChromatinBednar, J., et al . Nucleosomes, linker DNA, and linker histones form a unique structural motif that directs the higher-order folding and compaction of chromatin. Proceedings of the National Academy of Sciences 95 , 14173–14178 (1998) Fischle, W., et al . Histone and chromatin cross-talk. Current Opinion in Cellular Biology 15 , 172–183 (2003) Hewish, D. R., and Burgoyne, L. A. Chromatin sub-structure. The digestion of chromatin DNA at regularly spaced sites by a nuclear deoxyribonuclease. Biochem Biophys Res Commun 52 , 504-510 (1973) Kornberg, R. D. Chromatin structure: A repeating unit of histones and DNA. Science 184 , 868–871 (1974) Luger, K., et al . Crystal structure of the nucleosome core particle at 2.8 A resolution. Nature 389 , 251–260 (1997) ( link to article ) Noll, M. Subunit structure of chromatin. Nature 251 , 249–251 (1974) ( link to article ) Olins, A. L., & Olins, D. E. Spheroid chromatin units (v bodies). Science 183 , 330–332 (1974) Olins, D. E., & Olins, A. L. Chromatin history: Our view from the bridge. Nature Reviews Molecular Cell Biology 4 , 809–814 (2003) ( link to article ) Oudet, P., et al . Electron microscopic and biochemical evidence that chromatin structure is a repeating unit. Cell 4 , 281–300 (1975) Smith, C. L., & Peterson, C. L. ATP-dependent chromatin remodeling. Current Topics in Developmental Biology 65 , 115–148 (2005) Thomas, J. O., & Kornberg, R. D. Octamer of histones in chromatin and free in solution. Proceedings of the National Academy of Sciences 72 , 2626–2630 (1975) Van Holde, K. E. Chromatin: Springer Series in Molecular Biology (New York, Springer-Verlag, 1988) Van Holde, K. E., et al . A model for particulate structure in chromatin. Nucleic Acids Research 1 , 1579–1586 (1974) Wolffe, A. P. Chromatin: Structure and Function , 3rd ed. (San Diego, Academic, 1999) Woodcock, C. L. A milestone in the odyssey of higher-order chromatin structure. Nature Structural and Molecular Biology 12 , 639–640 (2005) ( link to article ) Woodcock, C. L., et al . Structural repeating units in chromatin. I. Evidence for their general occurrence. Experimental Cell Research 97 , 101–110 (1976)https://www.nature.com/scitable/topicpage/dna-packaging-nucleosomes-and-chromatin-310/DNA is Packed into a Mitotic Chromosome.DNA is Packed into a Mitotic Chromosome. Rate & Certify Rate Me... Comment DNA is Packed into a Mitotic Chromosome. The nucleosome, formed by DNA and histones, is the essential building block in this highly packed structure. Please provide your notes.https://www.nature.com/scitable/content/DNA-is-Packed-into-a-Mitotic-Chromosome-3497/Image: Dyads (homologous pair) 7.1: ChromosomesFor most of the life of the cell, chromosomes are too elongated and tenuous to be seen under a microscope. However, before a cell is ready to divide by mitosis, each chromosome is duplicated (during S phase of the cell cycle). As mitosis begins, the duplicated chromosomes condense into short (~ 5 µm) structures which can be stained and easily observed under the light microscope. These duplicated chromosomes are called dyads.When first seen, the duplicates are held together at their centromeres. In humans, the centromere contains 1–10 million base pairs of DNA. Most of this is repetitive DNA: short sequences (e.g. 171 bp) repeated over and over in tandem arrays. While they are still attached, it is common to call the duplicated chromosomes sister chromatids, but this should not obscure the fact that each is a bona fide chromosome with a full complement of genes.The kinetochore is a complex of >80 different proteins that form at each centromere and serves as the attachment point for the spindle fibres that will separate the sister chromatids as mitosis proceeds into anaphase. The shorter of the two arms extending from the centromere is called the p - arm; the longer is the q - arm. Staining with the trypsin-Giemsa method reveals a series of alternating light and dark bands called G bands. G bands are numbered and provide "addresses" for the assignment of gene loci.Image: The structure of a chromosome. A duplicated chromosome is composed of two sister chromatids, bound together at an attachment site called the "centromere." Hans Ris, University of Wisconsin, Madison, WI. http://www.nature.com/scitable/resource?action=showFullImageForTopic&imgSrc=/scitable/content/ne0000/ne0000/ne0000/ne0000/104572910/18336_2.jpgTo understand the answer we would have to understand some basics; these would help us comprehend the exact logic applied to arrive at a solution.First, for the students who are in a rush, I will provide the answer -One important thing that should be remembered here is, that even though the chromosome in a cell, that has passed the S phase, appears to be single or 1 (one) when viewed under a microscope, the genetic material has undergone duplication. Hence, even though the centromere appears to be single, it has double the amount of genetic material joined through cohesin (Cohesins hold sister chromatids together after DNA replication until anaphase when removal of cohesin leads to separation of sister chromatids Cohesin - Wikipedia), when compared to a centromere of a resting cell (non-dividing); therefore, separates into two in Anaphase (mitosis) and Anaphase II (meiosis).As a cell enters anaphase, cohesin Cohesin - Wikipedia is precipitously degraded, and the cell's sister chromatids separate to opposite poles of the spindle.Image source: http://slideplayer.com/slide/4018244/Image source: STUDYBLUE | Find and share online flashcards and notes from StudyBlue. Any subject, anywhere, anytime.i.e The human cell is diploid, with 2n = 46. In the prophase of mitosis, cells have __46__ chromosomes per cell, each with __2__ chromatid(s). In anaphase of mitosis, cells have __92__ chromosomes per cell, each with __1__ chromatid(s).There are 46 chromosomes in a human cell, and hence, 92 centromeres (or double the number of chromosomes in a cell i.e. 2 X 2n = 4n) at Anaphase I. [The first meiotic division ends with two cells each with the same number of chromatids as the parent cells but half the number of chromosomes; hence, Meiosis I is also known as reduction division.] But at the end of Telophase I, the two cells would have 46 centromeres and 46 chromatids equal to that of the parent cell but 23 (n) chromosomes.The catch in the second meiotic division or Meiosis II is that the homologous chromatids do not duplicate but merely separate. There is no S phase before Meiosis II.; whereas, in Anaphase II there are 46 (2n) centromeres and 46 (2n) chromatids in each cell. At the end of Telophase II, there will be 4 cells, each with 23 (1n) centromeres and 23 (1n) chromatids.There are 92 (4n) centromeres and 92 chromatids (4n) at Anaphase of Mitosis.Image: Vertebrate kinetochore ultrastructure (A) A schematic of a mitotic chromosome with paired sister chromatids the chromatid on the right is attached to microtubules and the chromatid on the left is unattached. The inner kinetochore, the outer kinetochore, the inner centromere and the fibrous corona, which is detectable on the unattached kinetochore, are highlighted. (B) Electron micrograph of a human kinetochore (image courtesy of Y. Dong and B. McEwen, State University of New York at Albany, USA). The micrograph represents a single slice from a tomographic volume of a high-pressure frozen mitotic cell and has been labelled as in a to highlight the key structural features of the kinetochore. Scale bar, 100 nm. © 2007 Elsevier McEwen, B. F., Dong, Y. & VandenBeldt, K. J. Using electron microscopy to understand functional mechanisms of chromosome alignment on the mitotic spindle. Methods in Cell Biology 79, 259–293 (2007). All rights reserved. http://www.nature.com/scitable/resource?action=showFullImageForTopic&imgSrc=/scitable/content/18070/10.1038_nrm2310-f2_full.jpgPeople who are well versed with the basics can skip the next part.Centromere - The centromere is easily visualised as the most constricted region of a condensed mitotic chromosome. Although the word "centromere" is derived from the Greek words Centro ("central") and mere("part"), centromeres are not always found in the centre of chromosomes. In fact, only so-called metacentric chromosomes have centromeres at their middle; in other chromosomes, centromeres are located at a variety of positions that are characteristic of each particular chromosome. With a few exceptions, eukaryotic chromosomes have a single centromere that ensures their accurate segregation during mitosis. Chromosomes that lack centromeres segregate randomly during mitosis and are eventually lost from cells. At the other extreme, chromosomes with multiple centromeres are subject to fragmentation if the centromeres become attached to opposite spindle poles by way of their kinetochores. http://www.nature.com/scitable/topicpage/chromosome-segregation-in-mitosis-the-role-of-242#Figure: Eukaryotic chromosome classification. On the basis of the location of the centromere, chromosomes are classified into four types: metacentric, submetacentric, acrocentric, and telocentric.© 2013 Nature Education Adapted from Pierce, Benjamin. Genetics: A Conceptual Approach, 2nd ed. All rights reserved. http://www.nature.com/scitable/resource?action=showFullImageForTopic&imgSrc=/scitable/content/ne0000/ne0000/ne0000/ne0000/113348580/46127_3.jpgAfter the M phase (the period in which mitosis, the process of nuclear division, occurs), the daughter cells each begin a new cycle by proceeding to interphase. Each stage of interphase (interval between nuclear divisions, beginning after cytokinesis and lasting until just before the beginning of prophase in the next round of mitosis) has a distinct set of specialized biochemical processes that prepare the cell for initiation of cell division (see figure below).Image source: The cell cycle, mitosis and meiosisImage source: Life Sciences CyberbridgeS phaseNow, in the S phase which follows the G1 phase, all of the chromosomes are replicated. Following replication, each chromosome now consists of two sister chromatids [either of the longitudinal subunits produced by chromosomal replication] (see figure below).Thus, the amount of DNA in the cell has effectively doubled, even though the ploidy, or chromosome count, of the cell, remains at 2n.[ploidy= Chromosome count, 2n if diploid, n if haploid. In humans, 2n=46 in diploid somatic cells, and n = 23 in haploid germ cells.]Note: Chromosomes double their number of chromatids post replication but the nuclei remain diploid as the number of centromeres and chromosomes remains unchanged. Hence, the number of chromosomes in the nucleus, which determines the ploidy, remains unchanged from the beginning to the end of the S phase.Image source: Life Sciences CyberbridgeMITOSIS OR M PHASEImage Source: Cell-Cell division and growthImage source: Life Sciences CyberbridgeAnaphaseAfter all of the chromosomes are aligned on the metaphase plate, each pair of sister chromatids splits at the centromere, separates and moves along the shortening spindle fibres to opposite sides of the cell. Now, the number of centromeres and chromosomes within the cell is doubled. Note that each separated chromosome has only one chromatid (either of the longitudinal subunits produced by chromosomal replication).MEIOSIS IMeiosis I segregates homologous chromosomes, which are joined as tetrads (2n, 4c), producing two haploid cells (n chromosomes, 23 in humans) which each contain chromatid pairs (1n, 2c). Because the ploidy is reduced from diploid to haploid, meiosis-I is referred to as a reductional division. Meiosis II is an equational division analogous to mitosis, in which the sister chromatids are segregated, creating four haploid daughter cells (1n, 1c).In meiosis, the chromosome or chromosomes duplicate (during interphase) and homologous chromosomes exchange genetic information (chromosomal crossover) during the first division, called meiosis I. The daughter cells divide again in meiosis II, splitting up sister chromatids to form haploid gametes. Two gametes fuse during fertilization, creating a diploid cell with a complete set of paired chromosomes.Image Source: Cell-Cell division and growthIn anaphase I, homologous chromosomes separate. Homologous chromosomes, each containing two chromatids, move to separate poles. Unlike in mitosis, the centromeres do not split and sister chromatids remain paired in anaphase I. Life Sciences CyberbridgeAt Anaphase I, the number of chromosomes and centromere remain to be 2 (n).So, the number of chromosomes is halved in each cell i.e. 1 (n), but the number of centromeres remains 2(n), in each of the two cells, after Meiosis I is completed.MEIOSIS IIAnaphase IIIn anaphase II, chromosomes divide at the centromeres (like in mitosis) and the resulting chromosomes, each with one chromatid, move toward opposite poles of the cell.Image source: Life Sciences CyberbridgeSource: Harvard’s Life Sciences CyberbridgeHence, at the end of Anaphase II, the number of chromosomes inside a cell is (n) but the number of centromeres and the number of chromatids is (2n). After meiosis II is completed, 4 cells are produced, each with 1 (n) number of chromosomes, 1 (n) number of centromeres, and 1 (n) number of chromatids.Edits and suggestions are always welcome. Feedback would be appreciated and genuine concerns are always acknowledged.
How is any degree of similarity maintained among the individuals of an asexually reproducing species?
What a fascinating question.What I personally find most interesting about this question was that, in trying to answer it, I kept discovering false assumptions I didn't know I had. So I'd like to thank you up front, Bill, for asking such a thought-provoking question.In my answer I'm going to suggest some ways similarity is maintained, but also try to pick apart some of the assumptions I think most of us carry without realising it.[Note: When we think of asexually reproducing species, we may also think of some fungi, such as yeast, or invertebrates, such as aphids, which have an asexual phase to their life cycles, but I think most people would have bacteria in mind with a question like this. I am going to focus mainly on bacteria, as we are more familiar with them and they are more 'obligately' asexual than species such as aphids.]Why do we think asexually reproducing species maintain similarity?This is obviously a fundamental assumption underlying the question, and yet it's difficult to say to what extent it it is true. We talk about Escherichia coli and Staphylococcus aureus, and because we are giving a name to a species, and because we understand bacteria to reproduce by producing identical, cloned daughter cells, we imagine the label describes static categories.But on the other hand, we know that evolution depends on replication, which entails mutation, and that an accumulation of mutations in the presence of selection factors leads to significant change - evolution. So it seems odd that we still have the same species of E. coli in our bowels as when we were born, and indeed the same species as everyone else on the planet. Why haven't they evolved to a different species?I think part of the answer is that when we compare bacterial species to familiar, sexually reproducing, macroscopic species, we are not comparing apples and apples. The definition of species is actually different. Two E. coli cells could be as different as donkeys and horses and yet we might still categorise them as one species. Why?The most common definition of a species barrier for sexually reproducing species is something like "unable to interbreed to produce fertile young". This definition is clearly not applicable to asexually reproducing species. And if we try to categorise them according to their genetic commonality, we're still not comparing apples and apples. Consider:Horses have 64 chromosomes and 2.7 billion base pairs [1].Humans have 46 chromosomes and 3 billion base pairs.[2]E. coli has 1 chromosome and 4.6 million base pairs [3].That's a difference of around three orders of magnitude in number of base pairs. Saying that two specimens were, say, 99.9 per cent identical might not mean the same thing for E. coli as it would for eukaryotic species.And in fact, the methods for comparing bacterial species are often even less likely to make this distinction. There are several competing methods of categorising organisms, and bacterial taxonomy tends to be more cladistic -- based on observable traits -- primarily because bacteria are studied for medical purposes. A bout of diarrhea may be caused by the introduction of virulent strains of E. coli into the digestive system, or by some closely related species, or by unrelated different species, or by an entirely novel strain that has evolved in the patient's own bowel.[4] But when you're trying to work out a diagnosis and treatment, you really only care about a handful of basic traits, not the academic distinction of whether a person's bowel flora have just given rise to a fascinating new species of bacterium. In diagnostics, you really just want to know whether it falls into the category that responds to this antibiotic or that one.So again, we call the rod-shaped bacteria in our bowel E. coli and imagine them to all be the same, but they are actually fairly diverse. They do evolve all the time. The E. coli long-term evolution experiment found some quite significant evolutionary changes over 60,000 generations, including one culture that developed the ability to use citric acid for aerobic respiration, an astounding development that is arguably an example of speciation in the laboratory.[5]There are actually many thousands of identified strains of E. coli, with names like Bc251 and O157:H7, and undoubtedly many, many thousands more we have not identified or characterised. The same is true for other bacteria. For example, Nylon-eating bacteria are a strain (species?) of Flavobacteria that have evolved to digest byproducts of nylon manufacture that did not exist prior to the 1930s. We only know about them because a group of Japanese scientists decided to study the bacteria in a pond that contained runoff from a nylon factory.If a farmer's sheep flock gave rise to a sheep that was essentially a different species, you wouldn't need a scientist to be studying the flock at the time in order for someone to notice it. But if the same happens in a bacterial population, usually nobody notices. For every new species of mosquito[6] we do notice, I bet there are at least 100 new species of bacteria we don't.After all, who is to say that the E. coli in our bowels today are the same species as those that graced the intestines of Tutankhamen, Alexander the Great, William Shakespeare, or even our own great grandparents? The E. coli genome wasn't published until 1997, and it's fair to assume human bowels are periodically recolonised by the 'latest' strains.And yet... despite all that I have said so far, it is still the case that the E. coli you and I carry are very similar. Simple experiments can identify them as E. coli despite the fact that they must be separated by millions of generations and thousands of kilometres. We find this remarkable. And yet in a sense we shouldn't. Why not?Why do we think asexually reproducing species would differ from us in their ability to maintain similarity?We tend to think of ourselves as distinct from asexually reproducing single-cell organisms, but consider this: We, and all multicellular organisms, are essentially colonies of asexually-reproducing single-cell organisms.The human body comprises around 10^13 (that is, ten trillion) cells. They are all notionally identical, having arisen from a single diploid cell (zygote) through mitosis, or asexual reproduction, in the same way bacteria multiply. Throughout our lifespan, most of these cells continue to replicate, some with great rapidity. For example, rodent intestinal epithelial cells have a turnover rate of 1.35 to 3 days.[7] Many trillions of asexual cell divisions have already occurred in your skin and intestines, as in mine.And yet, we all carry around the assumption that the cells of our body are genetically identical, bar a mutation here or there. If we think that of ourselves, why do we have different expectations for the 10^14 bacterial cells living in our bodies?[8]So one way similarity is retained among bacteria is that they clone their entire genetic sequence, just as we do during mitosis.How else is similarity maintained?In fact, despite our impression that our cells are all near-identical clones, over a human lifetime they do accumulate mutations. Many of these mutations are meaningless. Some result in isolated cell deaths. And some mutations can accumulate to erode similarity in function, potentially improving one cell line's short-term fitness at the expense of 'rival' cells. In multicellular organisms, the result is cancer.Cancer is a big problem for multicellular organisms, because it kills the colony. To continue reproducing indefinitely, the genome must escape the limited colony of the body (sexually reproduce) before the organism collapses. This is perhaps a more important 'purpose' of sexual reproduction than recombination; germ line cells are sequestered from replication, and recombination serves as a sort of error check each generation.[9] To this end, eukaryotic organisms have many different DNA repair mechanisms, which are a brake on at least some forms of genetic change, including but not limited to proto-cancerous mutations.Of course, these eukaryotic cell safeguards also apply to the few multicellular organisms that do not undertake sexual reproduction.But importantly, they also apply to asexually reproducing bacteria. The bacteria we find in the environment have been through several billion years of evolution, and usually billions of generations in their current environment. Their genomes are very finely adapted, with each gene being dependent on the function of other genes to survive. This highly interdependent system means that many mutations are simply noncompetitive. Even a potentially beneficial mutation may be outcompeted if it 'upsets the apple cart' by reducing the efficacy of traits the bacterium has evolved to depend upon. For example, if the aforementioned set of mutations allowing anaerobic respiration in E. coli occurred outside the lab, it might confer minimal advantage in the largely anaerobic atmosphere of the bowel, and side-effects of the mutations might render the cell line less competitive. This itself keeps an E. coli cell looking like an E. coli cell - because even significant genetic shifts tend to lead to a cell that looks much like all the others, if it is to survive in the same environment.And while cancer is not applicable to bacteria, bacterial cells do have DNA repair mechanisms, which may control some forms of mutation.Nonetheless, I think the ultimate answer to the question "How is any degree of similarity maintained among the individuals of an asexually reproducing species?" is: It isn't, at least not any more than similarity is maintained among the cells of sexually reproducing organisms.Thanks again for providing me the opportunity to answer such an interesting question.Notes[1] Wikipedia: Horse[2] Wikipedia: Human genome[3] Wikipedia: Escherichia coli: Genomics[5] James P. Nataro and James B. Kaper, Diarrheagenic Escherichia coli, Clin Microbiol Rev. 1998 Jan; 11(1): 142–201.[6] Zachary D. Blount, Christina Z. Borland, and Richard E. Lenski, Historical contingency and the evolution of a key innovation in an experimental population of Escherichia coli, Proc Natl Acad Sci U S A. 2008 Jun 10; 105(23): 7899–7906.[6] Wikipedia: London Underground mosquito[7] B. Creamer, R. G. Shorter and John Bamforth, The turnover and shedding of epithelial cells, Gut, 1961, 2, 110.[8] Of course, over multiple human generations there is good reason to hold that humans should be more evolutionarily conservative than bacteria. Our germ line cells are segregated, undergoing far fewer replications. But within the 50-100 years of a typical human life, an unimaginable number of cell divisions takes place. It's odd that we have different intuitive expectations for rates of change in that unimaginable number of replications than in the unimaginable number of bacterial replications that takes place over the same time.[9] Research presented in Elvira Hörandl, Meiosis and the Paradox of Sex in Nature has demonstrated that the sexual reproduction phase of the yeast life cycle can be triggered by oxidative stress, which can cause genetic damage. This could be why sexual reproduction appeared soon after the Earth's atmosphere became rich with biogenic oxygen - recombination was a way of eliminating oxidative damage. For a more detailed answer relating to this, see my answer to Why, within evolution, would it be of benefit to move to sexual reproduction?
- Home >
- Catalog >
- Life >
- Score Sheet >
- Basketball Score Sheet >
- Basketball Scoresheet >
- youth basketball score sheet >
- 2 Chromosomes And Mitosis Answer Key