How to Edit Your Name: The Wave Complete Study Guide Online Easily and Quickly
Follow these steps to get your Name: The Wave Complete Study Guide edited with accuracy and agility:
- Click the Get Form button on this page.
- You will be forwarded to our PDF editor.
- Try to edit your document, like signing, highlighting, and other tools in the top toolbar.
- Hit the Download button and download your all-set document for the signing purpose.
We Are Proud of Letting You Edit Name: The Wave Complete Study Guide Seamlessly
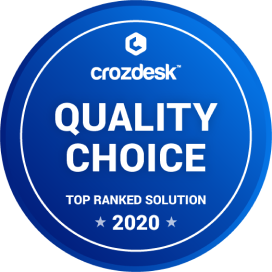
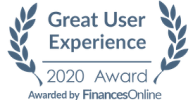
How to Edit Your Name: The Wave Complete Study Guide Online
When dealing with a form, you may need to add text, give the date, and do other editing. CocoDoc makes it very easy to edit your form with just a few clicks. Let's see how can you do this.
- Click the Get Form button on this page.
- You will be forwarded to CocoDoc PDF editor webpage.
- In the the editor window, click the tool icon in the top toolbar to edit your form, like checking and highlighting.
- To add date, click the Date icon, hold and drag the generated date to the field to fill out.
- Change the default date by modifying the date as needed in the box.
- Click OK to ensure you successfully add a date and click the Download button for the different purpose.
How to Edit Text for Your Name: The Wave Complete Study Guide with Adobe DC on Windows
Adobe DC on Windows is a must-have tool to edit your file on a PC. This is especially useful when you prefer to do work about file edit offline. So, let'get started.
- Click and open the Adobe DC app on Windows.
- Find and click the Edit PDF tool.
- Click the Select a File button and select a file to be edited.
- Click a text box to adjust the text font, size, and other formats.
- Select File > Save or File > Save As to keep your change updated for Name: The Wave Complete Study Guide.
How to Edit Your Name: The Wave Complete Study Guide With Adobe Dc on Mac
- Browser through a form and Open it with the Adobe DC for Mac.
- Navigate to and click Edit PDF from the right position.
- Edit your form as needed by selecting the tool from the top toolbar.
- Click the Fill & Sign tool and select the Sign icon in the top toolbar to make a signature for the signing purpose.
- Select File > Save to save all the changes.
How to Edit your Name: The Wave Complete Study Guide from G Suite with CocoDoc
Like using G Suite for your work to finish a form? You can integrate your PDF editing work in Google Drive with CocoDoc, so you can fill out your PDF without Leaving The Platform.
- Integrate CocoDoc for Google Drive add-on.
- Find the file needed to edit in your Drive and right click it and select Open With.
- Select the CocoDoc PDF option, and allow your Google account to integrate into CocoDoc in the popup windows.
- Choose the PDF Editor option to move forward with next step.
- Click the tool in the top toolbar to edit your Name: The Wave Complete Study Guide on the specified place, like signing and adding text.
- Click the Download button to keep the updated copy of the form.
PDF Editor FAQ
Why don't more physicists subscribe to pilot wave theory?
This is an important question, the best (simple) answer to which is probably: most physicists simply don’t know much about the pilot-wave theory, and much of what they think they know is wrong. To help illustrate this (and perhaps clear up some of the confusions) I wanted to address some of the common misconceptions that I saw in the various other answers to this question (and, indeed, in the original question itself). Apologies in advance for the length… there were many things I thought were worth commenting on when I first read through the page. OK, here goes:Original Question“It seems far more natural to accept non-locality than to give up determinism.”This idea, that one has to choose between determinism and locality, is a common and very unfortunate misconception, and is probably the most important factor explaining why most physicists have an unwarranted negative opinion about the pilot-wave theory. If it were true that one could only restore determinism to quantum mechanics by introducing some (relativity-violating) non-locality, I think physicists would be entirely right to reject this option. The price (of introducing a conflict with relativity theory, one of the bedrocks of modern physics, merely to avoid indeterminism) would simply be too high. But, in fact, this whole way of thinking about these issues is based on a completely wrong understanding of Bell’s theorem.It is correct that Bell proved (in 1964, with significant clarifications in subsequent decades) that any deterministic theory would have to be non-local in order to reproduce the (now-known-to-be empirically correct) quantum predictions. But Bell’s argument was a follow-up to the 1935 EPR argument, which had already established that determinism (i.e., a deterministic hidden variable theory) was required to avoid the non-locality that was already present in orthodox quantum mechanics. Most physicists have never studied or understood the EPR argument, but just accepted on faith what their teachers (who had also never studied or understood the EPR argument) told them: the argument is wrong/invalid and was refuted by Bohr. But Bohr’s reply is philosophical nonsense and there is nothing at all wrong with EPR’s argument. And anyway, it should be trivially obvious that orthodox quantum mechanics — which remember includes the wave function collapse postulate, which is manifestly non-local — is a non-local theory.(OK, here it is in a nutshell. Consider a pair of entangled particles — say, a pair of spin-1/2 particles in a state of total spin zero. This is a superposition of “particle 1 is spin-up and particle 2 is spin-down” and “particle 1 is spin-down and particle 2 is spin-up”. According to orthodox QM, as long as the pair is in this state, neither particle can be said to possess a definite spin orientation, up or down. Such definite spins only arise as a result of measurement/observation: if, for example, particle 1 is observed to be spin-down, the state of the two-particle system immediately “collapses” to “particle 1 is spin-down and particle 2 is spin-up”. Note in particular that particle 2 acquires, newly, a definite spin value immediately upon, and as a result of, the measurement of the spin of particle 1. Since the two particles could be arbitrarily far away, this constitutes an obvious kind of non-locality: particle 2 goes from having no particular spin, to being (say) spin-up, as a result of a physical intervention that happened perhaps a million miles away at the location of particle 1. EPR’s point was that, if we take seriously the orthodox/Copenhagen idea that the wave function provides a complete description of the physical states of the corresponding systems, this implies an unacceptable conflict between QM and relativity (which is supposed to prohibit “spooky” — i.e., faster-than-light — actions at a distance). EPR suggested, quite naturally and correctly, that to get rid of this spooky action at a distance, it would have to be the case that, in the above kind of example, particle 2 already possesses some definite but unknown spin which the observation of particle 1 simply makes known to us. This is a “hidden variable” view because it says that there are facts, about the physical states of the particles, that are not included in the wave function description. In this hidden variable kind of view, the wave function becomes at least partially about our knowledge, rather than a direct and complete description of physical systems, and so the collapse of the wave function need not imply any conflict with locality/relativity: it simply represents our finding out something (perhaps about a distant system) that we didn’t know before. There is a little more that needs to be said to establish that determinism is required to account, locally, for the kinds of correlations QM predicts in the standard EPR type scenario; but this is actually pretty obvious once the stuff I’ve said is grasped.)Anyway, given the EPR argument, it is quite misleading to say that Bell proved that nonlocality is the price one has to pay to restore determinism. What he actually proved (and, for what it’s worth, this was Bell’s own view about what he had proved, as he makes beautifully clear in virtually everything he wrote in the decades after his original 1964 paper) is that the one possibility for a local theory that had been left open by EPR (namely, a deterministic hidden variables theory) couldn’t restore locality. That is, even deterministic hidden variables theories have to be nonlocal to make the correct predictions; you can’t actually restore locality (to the already and obviously non-local ordinary QM) in the way EPR had suggested (namely, by adding hidden variables). That’s what Bell really proved: non-locality is an inevitable consequence of the quantum mechanical predictions — i.e., we now know, from the various experimental tests of the relevant quantum predictions (notably the three beautiful experiments done last year) that non-locality is simply a fact of nature that any viable theory will have to include.There is some controversial stuff there that people will have to think through in some detail. My goal here is obviously not to provide a rigorous version of the arguments, but instead just to point out that it is a mistake to think that, in deciding between the pilot-wave theory and ordinary QM, we are deciding between a theory that is non-local but deterministic, and one that is local but indeterministic. In fact we are deciding between two non-local theories, one deterministic and one not. I actually don’t think determinism is very important, so if it were true that the only relevant difference between the pilot-wave theory and ordinary QM was that one is deterministic and one isn’t, I wouldn’t have a strong preference and probably wouldn’t care about this whole topic much. As I’ll elaborate more below, this is not the only relevant difference between the theories and I think there are strong reasons to prefer the pilot-wave theory. But my point here is just that recognizing that ordinary QM is already, like the pilot-wave theory, non-local, radically changes the cost-benefit analysis at play here. I think this is actually the fundamental reason that more physicists don’t like, or at least respect and appreciate, the pilot-wave theory: they mistakenly believe that ordinar/orthodox/textbook QM is a local theory, so that the non-locality of the pilot-wave theory is some kind of physical aberration introduced for purely philosophical reasons.“…the need to account for an observer disappears…”This is true and really captures the essence of the difference between ordinary QM and the pilot-wave theory. The point, if you like, of the pilot-wave theory is not really to restore determinism, but to eliminate the aspects of ordinary QM that Bell called “unprofessionally vague and ambiguous” — namely, the ad hoc rules associated with observation/measurement. In particular, according to ordinary QM, the wave function of a system evolves in accordance with the Schroedinger equation, except when a measurement occurs, in which case something entirely different and inconsistent occurs instead. The problem is not so much that two different things can happen, but rather that the rule for what happens involves concepts like “measurement” or “observation” that seem too anthropocentric to appear in the supposedly fundamental laws of physics. The real virtue of the pilot-wave theory (in contrast to textbook QM) is that it just posits fundamental laws — for how the wave function and the particles behave — and these apply, all of the time, without exception. There are some other “alternative” theories, such as the spontaneous collapse theory of Ghirardi, Rimini, and Weber (GRW) and Pearle, which have this same virtue and are therefore very highly respected by the very same people who like the pilot-wave theory — even though these other alternative theories are not deterministic.“…as does the need for the bizarre notions of superposition and collapse…”Well, “superposition” is definitely still part of the pilot-wave theory (just as it is, totally unproblematically, a part of, say, classical electromagnetism). What the pilot-wave theory eliminates is not superposition per se, but rather the conceptual contortions associated with superposition in orthodox QM. For example, in the pilot-wave theory, the wave function of a particle may be in a superposition of being on the left and being on the right. But the particle itself has some definite location, either on the left or on the right. The wave is in a superposition and the particle has a definite position. I submit that there is nothing the least bit weird or problematical about that.Regarding collapse, it is both true and not true that the pilot-wave theory gets rid of wave function collapse. What it does get rid of is the collapse-of-the-wave-function as a dynamical axiom of the theory. According to the pilot wave theory, the wave function of the universe obeys Schroedinger’s equation always, end of discussion. It never collapses, period. But one of the more important (and least known) virtues of the pilot-wave theory is that it allows one to define the wave function of a sub-system (e.g., a single particle). Consider for example a two-particle universe with universal wave function S(x,y). In the pilot-wave picture, a complete description of the state of the universe would include not only the wave function S(x,y) but also the locations, X and Y, of the two particles. Anyway, one can then define the so-called “conditional wave function” for the first particle: S1(x) = S(x,Y). That is, the thing which one can naturally regard, in the pilot-wave theory, as “the wave function of the first particle” is just the wave function of the universe evaluated at the actual location of the other particle. Anyway, the incredible and beautiful thing is that the fundamental dynamical axioms (for S and X and Y) turn out to imply — yes, imply — that these one-particle (“conditional”) wave functions act in exactly the multiplicity of ways described by ordinary QM: they obey a one-particle Schroedinger equation when the systems are suitably decoupled, and they collapse when there is the kind of coupling that would ordinarily be described as a measurement. So instead of saying that the pilot-wave theory eliminates the collapse of the wave function, it would probably be better to say that the pilot-wave theory explains the textbook formalism, including the collapse postulate, by positing a set of ontological and dynamical axioms which (unlike textbook QM itself, with its obviously ad hoc special measurement postulates) can be taken seriously as a candidate fundamental theory.“…it might be more compatible with relativity than other interpretations…”I don’t think this is the case. The non-locality is very difficult (but perhaps not impossible) to reconcile with relativity. The crucial point here for most interested people, though, will be this: despite the common misconception to the contrary, the pilot-wave theory is not less compatible with relativity than standard/textbook/orthodox QM.(The pilot-wave theory may, in some very subtle sense, be less compatible with relativity than the spontaneous collapse theories mentioned above. And proponents of Everett’s “many worlds interpretation” will claim that that interpretation is entirely compatible with relativity and hence far superior to the pilot-wave theory and any other option. But Everett’s theory is a very special case that raises a number of difficult issues that its proponents need to do a much better job addressing before such claims can be taken too seriously.)Thad Roberts’ reply to the original question is excellent and I agree with almost everything he wrote. (Actually I only found this page because a Bohmian friend suggested I take a look at this one excellent response to the original question.) Still, there were a couple of things that I thought were either not quite right or deserved a bit of further elaboration/clarification…“…in addition to the Schroedinger equation … Bohmian mechanics [aka, the pilot-wave theory] is completed by the specification of actual particle positions, which evolve (in configuration space) according to the guiding equation.”The parenthetical “in configuration space” is a little confusing/misleading. It is true that the wave function (whether in the pilot-wave theory or in ordinary QM) is a function on configuration space. That means the wave function of the universe cannot be understood as an ordinary field (like the electric and magnetic fields of classical E&M) that lives in physical, three-dimensional space. So the wave function is quite weird and I think this is a very serious issue for any quantum theory in which the wave function plays a role. Note: it is an especially serious issue for Everett’s interpretation, where the wave function is supposed to be all there is! But one of the nice things about the pilot-wave theory, by contrast, is that one can regard the wave function as (mysterious, yes, but) in the background — perhaps even more akin to a law than a physical field. The ordinary physical world, in the pilot-wave theory, is not supposed to be “made of wave function”; instead, the trees and tables and cats and planets and galaxies of the physical world are, in the pilot-wave theory, made of the particles. And this is perfectly coherent precisely because, unlike the wave function, the particles just live and move around in ordinary, three-dimensional physical space. So the suggestion that the particles evolve “in configuration space” is puzzling and perhaps just plain wrong. It is of course possible to represent the positions of N particles in 3-space, with a single point in a 3N-dimensional configuration space. But the particles themselves should be understood to live and move in 3-space. Otherwise one loses (and loses pointlessly) one of the main things that makes the pilot-wave theory superior to other options (such as the Everett interpretation): it provides a straightforwardly coherent microscopic account of a world whose gross (coarse-grained) structure corresponds perfectly to the kind of world we observe in ordinary direct perception of tables, trees, etc.On a related topic, Thad Roberts notes that one of the (unfair and misguided) criticisms of the pilot-wave theory is that“… the model suggests that there is something called configuration space, asserting additional variables and creating a dualism almost Platonic in scope.”It may be true that some physicists reject the pilot-wave theory because they think it requires the introduction of configuration space. But I doubt this. Configuration space is already familiar to physicists from classical mechanics, and I think most physicists are aware that the quantum mechanical wave function is (mathematically) a field on configuration space. So if they cannot stomach a field on configuration space, they would have to already reject ordinary quantum mechanics. That is, as Thad Roberts correctly notes elsewhere in his reply, the “pilot-wave field” in the pilot-wave theory is just the ordinary wave function of ordinary quantum mechanics; so to whatever extent it is metaphysically problematic to have such a thing in one’s theory, this can hardly be a valid reason for rejecting the pilot-wave theory in favor of ordinary QM. (I might have misunderstood Thad Roberts’ point here, but it sounds like he was conceding, to the critic, that the pilot-wave theory does indeed introduce configuration space, but then denying that this is as problematic as the critic suggests. But I think the premise is just wrong. The pilot-wave theory does not at all introduce configuration space; it merely inherits it from the wave function that is already present in ordinary QM.)“In order to accept th[e orthodox] interpretation [rather than the pilot-wave theory] we have to do more than abandon our notion of a particle—we have to accept that this magic is truly just that—magic. We have to accept that it really is impossible for us to ever have intuitive access to the process that causes photons, electrons, etc., to form interference patterns in the double-slit experiment—that it is impossible for us to comprehend, understand, or ever know what’s really going on during these experiments.”I agree that there is an element of magical thinking involved in trying to understand what is happening in (e.g.) the 2-slit experiment according to orthodox QM. But I think this overstates the extent to which the orthodox picture is counter- (or anti-) intuitive. Most physicists think that it is easy to intuitively understand how the particle can go through both slits — the “particle”, they say, is not really a particle (in the literal, classical, pointlike-object-with-a-sharply-defined-position-following-a-definite-trajectory sense). Instead, what we call “particles” are really smeared-out wave-like blobs. And it is perfectly clear, intuitively and otherwise, how a smeared-out wave-like blob can spread out over a large region of space and hence (e.g.) partially go through each of several slits. The problem, then, is not specifically with understanding how the particle can be in two places at once, but is, instead, with reconciling the wave-like blob picture (which easily explains interference, just as in classical wave mechanics) with the (literal, pointlike) particle picture (which seems to be required by the experimental fact that each “particle” eventually registers at the detection screen at a sharp location. In the textbook theory, the transition from “smeared-out wave-like blob” to “literal point-like particle” is precisely the collapse of the wave function and so at the end of the day the only problem with understanding the 2-slit experiment from the point of view of ordinary QM is just the problem of the collapse postulate, considered as a dynamical axioms, being “unprofessionally vague and ambiguous”. The difficulty, that is, is providing a uniform and consistent account which does not rely on ad hoc rules involving fuzzy, anthropocentric notions.I wanted to stress this point because sometimes critics think that the people who are attracted to the pilot-wave theory are attracted to it because of its ontology of (literal point-like) particles. But here I think the critics are just wrong. I guess there is something terminologically convenient in the fact that, in the pilot-wave theory, one doesn’t have to constantly put scare-quotes around the word “particle”. (But actually this also causes a lot of terminological trouble, because, according to the pilot-wave theory, a “particle” — for example, a single electron — is two things: a literal pointlike particle and an associated wave, described by a wave function obeying the Schroedinger equation. So it can actually be quite difficult even to convey the nature of the ontology postulated by the theory, without departing too far from standard word usage.) But the truth is that no serious person who likes the pilot-wave theory likes it because of some kind of ridiculous, old-fashioned, dogmatic insistence that “particle should mean particle”. Everybody perfectly well understands the empirical basis for the hypothesis that (what we call) “particles” are really not (literal pointlike) particles at all, but are instead some fuzzy cloudy wavy things. And everybody is perfectly open to candidate theories that make such a picture (of electrons, etc., as fuzzy cloudy wavy things) precise (by articulating for example the exact equations that the fuzzy cloudy wavy things would have to obey to account for the detailed experimental data. The problem is, ordinary QM is not such a theory (because instead of — or, to be more generous, in addition to — some precise equations, we have ad hoc hand-wavy imprecisely-defined rules about which equations apply when). So the appropriate reason to like the pilot-wave theory is not that one finds (literal pointlike) particles metaphysically appealing and fuzzy cloudy things unappealing, but rather just that one can formulate a sharp and clean physical theory involving the particles. If/when somebody can figure out how to formulate a sharp and clean theory that has some fuzzy cloudy blobs instead of particles, I will be very interested in that as well.“If every particle were to follow completely identical trajectories, then they would all end up at one spot, creating a single bright spot on our photographic plate (or the wall). In Nature, this is not a real possibility for photons because the substrate of the vacuum is composed of interactive quanta. For two particles to follow identical trajectories, identical trajectories must exist. They don’t because the vacuum is not static. The quanta that compose the vacuum are constantly mixing about in configuration space. In quantum mechanics, the best information about available four-dimensional trajectories is given by an equilibrium distribution because quantum mechanics explicitly codifies a vacuum that is in a state of equilibrium. The inherent mixing of the vacuum explains why extremely precise information about a trajectory in the familiar four dimensions can at best be described statistically, or probabilistically.”I think there is a much simpler explanation for why (even though the pilot-wave theory is deterministic) all the particles in a 2-slit experiment don’t follow the same trajectory and hence land at the same spot on the screen: even if the wave function (of each successive “particle” that is shot through) is exactly the same, the initial positions of the (literal pointlike) particles won’t be.It’s perhaps true that, if this is all one says, one might be accused of giving the false impression that better future technology might someday allow us to more carefully control the initial positions of the particles, and then we would be able to arrange for all the particles to land exactly where we want (all in the same spot or whatever). This is almost certainly not the case though. The pilot-wave theory very strongly suggests that our control of the initial positions of the particles within their guiding waves can never be improved; this is closely related to the theory’s explanation of the Heisenberg uncertainty principle. In the orthodox theory, this principle is really more of an “objective indefiniteness principle” since it is not merely about our ignorance of something which is in reality definite. In the pilot-wave theory, one can understand “uncertainty” more literally. But, as it turns out, this uncertainty remains in some sense absolute: although the uncertainty principle describes the extent of our ignorance about things which are, in reality, perfectly definite, it is actually an implication of the fundamental dynamical laws of the theory that we could never acquire information beyond the limits described in Heisenberg’s formula. This is actually not as weird as it sounds. The point is just that, while we can always acquire more information about (say) the position of a particular particle, the physical intervention required affects the (“conditional”) wave function of that particle, which in turn makes the particle move, subsequently, differently than it would have absent the intervention. So, in particular, some information about the momentum of the particle may be lost. As is so often the case, the pilot-wave theory doesn’t really eliminate important quantum mechanical concepts (here, the idea of the disruptive effect of measurement and its relation to the Heisenberg principle) but instead explains and demystifies them.Anyway, there are some interesting issues in the background here, but my point was just to note that the explanation of quantum randomness, according to the pilot-wave picture, is really quite simple and need not bring in fancy ideas like zero-point fluctuations of the quantum field: as a matter of fact, one cannot perfectly control the initial positions of the particles one works with, and the different initial positions within some ensemble of otherwise identically-prepared systems will lead, via the deterministic dynamics, to different final positions.Ed Caruthers“The problem with pilot wave and other 'hidden variables' theories is, they don't lead anywhere. As the Wikipedia article says, the math is the same and the experimental tests are the same. There's no new prediction. There are no new experiments to do. As Joshua Engel said, it's an interpretation or explanation.”The math is not all the same. There are some elements in common (such as the Schroedinger equation and, at the end of the day, the formulas for empirical probabilities) but also a lot of differences: for example, ordinary QM has the collapse postulate (and other associated measurement axioms) whereas no such thing appears in the basic axioms of the pilot wave theory; and similarly, the pilot-wave theory includes the guidance equation (describing how the particles move under the influence of the wave function) whereas obviously there is no such equation in ordinary QM. It is true that the empirical predictions are the same at the end of the day. But there are two important points to make about that.First, and contrary to whatever cracker-jack-box philosophy one might have picked up from physics teachers, this does not make them the same theory — any more than, say, Ptolemy’s and Copernicus’ ideas about how the solar system worked were the same theory. Ordinary QM and the pilot-wave theory (and GRW and Everett and …) tell radically different stories about what is going on, physically, in the world. At most one of them can be correct. If you said “because these theories all make the same empirical predictions, it is, at least at present, impossible to decide on the basis of experiment which one is right, and it is therefore a waste of my time to worry about this” I wouldn’t have anything negative to say in response. Of course, I might note that people could have said (and did say) the same thing about all kinds of other similar disputes (between at-present empirically equivalent candidate theories) in the past, and it’s surely a good thing that at least some people (Galileo and Boltzmann especially come to mind here) did find it worth their time to worry about it. Still, different people have different interests and different areas of expertise and skill and that’s good. I don’t think everybody should worry about the Copenhagen vs Bohm debate, or even take a position on it. But people should more widely recognize it as a legitimate debate, and definitely should have enough sense to recognize the options as genuinely distinct theories.Second, and as others have pointed out, the fact that the theories make identical predictions can (for obvious symmetry reasons) never provide a basis for preferring one to the other. To say “the pilot-wave theory makes no new predictions, so I’ll reject it in favor of the orthodox theory” is apparently to prefer the orthodox theory for some reason that has nothing to do with the empirical superiority of that theory: because the person in question happened to learn about it first, or because it achieved orthodox status first, or because it will help them get along with colleagues better, or who knows what. The point is, people who criticize the pilot-wave theory for not making any new predictions should be willing to recognize that the same fact can be expressed more neutrally this way: the pilot-wave theory is exactly as successful, at correctly predicting the results of experiments, as ordinary QM. And they should therefore assess the status of the two theories accordingly.“…I find that Occam's razor saves me spending time on theories that assume there are multiple extra things, that I can't see, can't measure, can't test, and can't use. Pilot wave theory assume there are "real" electrons that "really" have exactly and simultaneously defined position and momentum, and that "really" behave like point charges, even though that's not what experiments show. So then there has to be a new thing, a pilot wave that somehow distributes large populations of "real" electrons so they'll behave, in aggregate, like experiments turn out, even when the experiments are done very slowly, with very small numbers of electrons. The pilot wave is a new thing, but the theory doesn't really let us use it.”This, I think, is just based on confusion/ignorance about what the pilot-wave theory says. The pilot-wave in the theory is not a new thing at all; it is just the wave function, familiar from ordinary QM, obeying the familiar Schroedinger equation. It is true, actually, that the wave function can’t be measured. (If I hand you a particle in a box, which I have prepared with a particular wave function which I know, you cannot experimentally determine what the wave function is.) But this is no more a problem for the pilot-wave theory (if it is a problem at all) than it is for ordinary QM. The pilot-wave theory does posit something “new” in the (literal pointlike) particles, but these can be measured (if you want to know the position of a particle, just measure it and find out) and they are not invisible: as mentioned above, literally what you see when you open your eyes and look around, according to the pilot-wave theory, is (big macroscopic collections of) the particles. As to testing, the pilot-wave theory makes (as I thought Ed had conceded) the same empirical predictions as ordinary QM, so it can be (and has been) tested to exactly the same degree, and with exactly the same level of empirical success and support, as textbook QM. Is the theory useful? I find it very useful as a way of understanding how the world might actually work.Finally what about Occam’s razor? If the pilot-wave theory makes the same empirical predictions, but has to posit not only the wave function but also the particles, aren’t we getting the same bang for more bucks, a worse deal? The problem with this argument is that ordinary QM also posits more than just the wave function obeying Schroedinger’s equation. To begin with, in ordinary QM, the wave function obeys Schroedinger’s equation only some of the time. There are extra rules for what happens at other times. And then, at least in Bohr’s understanding of the theory, it is only the microscopic realm that is to be described in terms of wave functions. There is, in addition, the whole classical macroscopic realm (necessary, in Bohr’s view, to account for our ordinary perception of the familiar world). So which is simpler, in Occam’s sense — a theory that posits a universal wave function and a bunch of particles with one simple equation describing the dynamics for each of those things — or a theory that posits wave functions for microscopic systems (whatever exactly that means) with several different dynamical rules that apply in different kinds of situations depending on how/whether the systems are interacting with one another and/or the other, classical type of system that the theory says exists and which also has different dynamical evolutions depending on how/whether the classical systems are interacting with microscopic systems (and let’s just leave aside the whole question of how any of this is even remotely coherent at all since micro and macro are not two different kinds of systems, but instead just two different perspectives on, or two different sizes of, the very same one kind of physically real system)? Of all the reasonable answers that could be given, the one that is most generous to the critic of the pilot-wave theory is: it’s not clear which theory is really simpler in the Occam’s razor sense.Joshua Engel“The various interpretations all try to give up one of "local", real", "definite", or "deterministic" in order to preserve the others, thus preserving at least a modicum of their intuition.”I already addressed this (way) above, but it deserves repeating. There is no (empirically viable) theory that is local. (Perhaps I should say “with the possible exception of Everett” but it really pains me to say that without going into a long tirade about how, since Everett doesn’t even involve any physical stuff in 3D space at all, the claim that it is a local theory is at best nonsense. So nevermind.) Let’s put it this way: if you want to criticize the pilot-wave theory for being non-local, the entrance fee is displaying your own preferred local (and empirically viable) alternative theory. If you claim that your preferred local and empirically viable alternative theory is ordinary QM, you will be sent home to do your homework (i.e., review the EPR argument and the chapter of the QM book about the collapse postulate). This idea, that non-locality is introduced specifically by the attempt to restore “reality” or “definiteness” or “determinism” to QM is simply wrong and it is an embarrassing tragedy that so many people believe it. The truth is that, to whatever extent you want any of these things (“reality” or “definiteness” or “determinism”) you can have them, for free.“But really, the predominant interpretation is no interpretation at all, the "shut up and calculate" school, which gives up on all of the intuitions at once rather than risk being misled by them.”This may be true as a matter of sociological fact, but that doesn’t make it sensible — not if “shut up and calculate” means “nobody should worry about and work on these questions” as opposed to just “I’m not going to worry about or work on these questions” or “You, dear student, should remember to set these worries aside and actually learn how to use QM”, both of which are perfectly reasonable.I’ll just repeat that I’m glad that, in earlier historical episodes, people like Galileo and Boltzmann rejected the “shut up and calculate” attitude (which, yes, was explicitly pushed by scientific losers both in regard to the question of whether the Sun or the Earth was really in the center of the solar system, and in regard to the question of whether atoms existed).“Without many huge wins to its credit, there's no particular drive for people to pick up the pilot wave theory.”I think it is true that the pilot-wave theory has not produced many “huge wins” in the sense meant here. Indeed, maybe someday it will; or maybe not. I don’t know. But it’s a better theory than textbook QM either way. But the point I really wanted to make here is that there is one “huge win” that the pilot-wave theory did lead to — that is, one universally-recognized major theoretical discovery that was directly stimulated by the pilot-wave theory, but which people don’t know of as such. This is Bell’s theorem, which is somewhat ironic sinse Bell’s theorem is so often mis-used as rhetoric against the pilot-wave theory. But the actual history is like this: Bell had been dissatisfied with Copenhagen QM since his student days but believed his teachers who told him that the possibility of a better, “hidden variable” type theory had been completely refuted by von Neumann. Then at some point after 1952, Bell stumbled across David Bohm’s 1952 papers presenting the alternative pilot-wave theory. He was profoundly moved by the reasonableness of the theory and in particular by the fact that it accomplished precisely what von Neumann had supposedly shown to be impossible. His first paper on quantum foundations (written in 1964 but not published, due to an editorial accident, until 1966) provided an analysis of what had gone wrong in von Neumann’s argument.But despite admiring the pilot-wave theory, Bell was also troubled by its nonlocality. He knew, of course, that EPR had shown that determinism was required if one was going to restore locality to QM, so he started thinking: could one have a theory that was deterministic (like Bohm’s) and shared its various other virtues (like avoiding all the subjective reference to observation and measurement in the dynamical postulates) but which also restored locality as in EPR’s fantasy? Undoubtedly he toyed with this question for a while, started to become increasingly skeptical about its being possible, and finally turned toward proving that it was impossible — which is precisely what he did end up proving and publishing in 1964.So, perhaps Bell’s theorem should count as a “huge win” for the pilot-wave theory.Paul Mainwood“Let's start with the hard nosed variety of physicist. An initial conversation is likely to go something like this:A: "Hi, I want to explain a new approach to quantum mechanics to you!"B: "OK, but I'm pretty busy, so can you just tell me how this new approach will impact any of my work?"A: "Not in any way! In fact, by design, pilot wave theory reproduces all the predictions of regular quantum mechanics. It's an interpretation rather than a real new theory."B: "OK, so why would I want to use this approach? Perhaps it's easier to calculate with? Like Feynman's path integral approach is (sometimes)?"A: "No, we've not found any problems which become easier. Actually, most are made slightly harder. But people will soon get used to it once we rewrite all the textbooks to use Bohmian language, and that won't take too long."B: "Erm, yes good luck with that. Does it suggest any extensions that might be interesting -- to field theory, relativistic versions and so on?"A: "Well, we don't know. It's actually quite hard to align it with relativity, because it's explicitly non-local. There's work being done, but it's in early stages. So, we haven't got a fully working relativistic theory yet, and the field theory versions don't work at all."B: "OK, great to meet you, I'm going to talk to someone else now."This made me smile, because it’s a pretty accurate summary sketch of many conversations I’ve had over the years. Still, it’s worth noting that physicist B here is a bit of a narrow-minded prick. I mean, would the conversation have been substantially different if A had been another professor from down the hall, or maybe the next department over, offering to explain to B (I dunno, say) the exciting new LIGO detections of gravitational waves, or some hot new discovery from biochemistry or paleontology? If B’s attitude is “if it’s not going to impact my current research project, I don’t care and don’t want to know”, he would apparently reject all these other things as well as the pilot-wave theory. Frankly I have a more positive and optimistic opinion about scientists in general. Yes, sometimes they’re too busy or too obsessed with their own research, but in general they are thoughtful people who enjoy learning about important scientific developments and issues, in the name of being well-informed and well-rounded and/or because it’s just interesting and fun. I think the real reason most physicists aren’t interested in the pilot-wave theory is that they think they know it’s wrong. But as I’ve explained, they’re simply wrong about that.BTW, it is completely wrong to say “the field theory versions don’t work at all.” There are pilot-wave type field theories that work fine. The problem is that these theories make the proper relativistic empirical predictions, but are not really relativistic theories “in their bones”. But this is just the nonlocality story over again. Orthodox “relativistic quantum field theory” is also not relativistic in its bones. (It, too, just like ordinary non-relativistic QM, requires collapse/measurement axioms that don’t play nice with relativity. The textbooks, however, are written in a way that conveniently suppresses this, e.g., by focusing almost exclusively on how to calculate S-matrix elements.)“But what happens if you have a more complex system (e.g., two particles)? Then the space in which the pilot wave evolves and the particle is guided is a six dimensional configuration space, which can no longer be identified with real space. And this gets worse every time an extra degree of freedom is added. For realistic systems, you end up with a billion dimensional configuration space, across which a pilot wave is guiding a "particle" that represents the whole system. This looks much less like a clear and simple ontology…”As I have said above, I agree that it is hard to understand the wave function for a generic N-particle system since this is something like a field, but on 3N-space rather than the usual 3-space. But this is no more a problem for the pilot-wave theory than it is for ordinary QM. In ordinary QM, our only description of microscopic systems is the wave function, and Bohr at least insisted (contra EPR) that this description was complete. Of course, it is hardly clear what exactly he meant by that. Probably he did not mean that we should regard the field-on-3N-space as a direct and literal description of the physical state. But if the wave function is all we’ve got, then that’s really our only option, other than just confessing that we do not yet know how to provide a direct and literal description of the physical states. (But Bohr didn’t mean that either, since that’s essentially what EPR were saying!) The point is, if you think it’s a legitimate scientific goal to try to provide a direct and literal description of microscopic physical states (and if you don’t think that, I think you’re crazy) then the pilot-wave theory should be recognized as perhaps the best available option: ordinary QM provides either no such candidate description at all, or a totally unbelievable and incomprehensible one, depending on how you understand it, whereas the pilot-wave theory provides a description that at least half makes perfect sense. What I mean, again, is that while the status of the wave function is mysterious, the other part of the ontology — the particles — is completely unproblematic and clear.So while I think it is valid and important to admit the puzzling status of the wave function (in any theory that posits it), I think Paul ends up underestimating the extent to which the pilot-wave theory provides a “clear and simple ontology” for one of the same reasons I mentioned above: the particles are just (literal pointlike) particles moving around in 3D space; one should not think of them as a single “marvelous point” in configuration space. The simple fact that the pilot-wave theory allows you to understand the world as being made of particles moving around in ordinary 3D space (with also some admittedly mysterious object in the background choreographing their motion), with familiar objects like tables and trees and cats and planets and galaxies being basically just huge collections of particles, should allow one to appreciate the ontological clarity of the theory… at least relative to any other extant option.“Pilot wave selects a preferred position basis (rather than, say, momentum). Why? This is an asymmetry that does not appear in classical QM, but pilot wave simply "writes in" a privileged status for position. This move is usually justified with a hand-waving argument that all measurements eventually come down to position (e.g., position of a pointer).”In terms of the evolution or representation of the wave function, there is no preferred basis. The universal wave function just obeys the Schroedinger equation, same as in ordinary QM, and you can work in whatever basis you want. (And anyway the Schroedinger equation already fails to respect the position/momentum symmetry I think Paul has in mind, at least for most/realistic Hamiltonians.) There are some subtle sorts of asymmetry of the sort I guess Paul means — e.g., what is ordinarily called a “position measurement” will simply reveal the pre-existing position of a particle according to the pilot-wave theory, but what is ordinarily called a “momentum measurement” will not in general simply reveal the pre-existing momentum (mass times dX/dt) of the particle. But it would be difficult to point to the place where “a privileged status for position” has been “written in” to the dynamics!The point about “all measurements eventually com[ing] down to position (e.g., position of a pointer)” is not hand-waving and isn’t an attempt to justify some kind of symmetry-breaking of the sort Paul seems to have in mind. Instead it’s part of the argument for the empirical equivalence of the pilot-wave theory and ordinary QM: it’s relatively easy to see that the pilot-wave theory should generate the same statistical predictions as ordinary QM for the positions of things; once you realize that even what are called “momentum measurements” or “spin measurements” also ultimately come down to the position of something (a pointer, or a flash on a screen behind a Stern-Gerlach magnet, or whatever) it is clear that the pilot-wave theory must generate the same statistical predictions as ordinary for every type of measurement.“…the pilot wave theory simply ignores the "action-reaction" principle, where if something is acted on by a field/particle/body, it "acts back" in analogy to Newton's third law. The particle is acted on by the pilot wave, but the pilot wave is not acted back on by the particle.”A literal violation of Newton’s third law would imply a violation of momentum conservation. The pilot-wave theory (which again makes the same empirical predictions as standard QM) certainly doesn’t predict such a thing. What Paul means here is that the theory violates some vaguely-defined analog to or extension of Newton’s third law. Before I could know whether this is really a problem, I would have to know exactly what this analog/extension says, and I would want to know what evidence there is for it. Since I think we agree that there is no experiment done to date for which the pilot-wave theory makes an incorrect prediction, it seems clear that it would be very difficult to establish that (i) this analog/extension principle is true, and (ii) the pilot-wave theory violates it.Still, I understand the spirit of the point and will confess that I also find it slightly weird that the wave function evolves autonomously, without influence from the particles, whereas the particles are very much influenced by the wave function. Maybe we just have to learn to live with weird things? (Everybody always says that about QM.) Or maybe we can come to grips with it. I have suggested elsewhere that perhaps the wave function is actually just a kind of indirect, abstract description of some other thing or things (maybe a whole set of single-particle guiding waves?) and the extended/analog action-reaction principle will ultimately be found to be respected once we sort out what we are actually talking about. Others have suggested that the wave function, in the pilot-wave theory, should not be understood as a physical field which pushes and pulls the particles the way E and B fields push and pull electrons (and note this idea of a physical field pushing and pulling on the particles is already seriously problematic since the field doesn’t even live in the same space where the particles live) but should instead be understood as more like a law, something more analogous to the Hamiltonian in classical mechanics. Then it would certainly be less puzzling that the particles don’t affect it.A final point is this: what better alternative do you propose? In the context of the original question here, it seems rather absurd to criticize the pilot-wave theory for failing to respect this analog/extended action-reaction principle, when the overwhelming majority of the physicists who subscribe, instead, to orthodox QM believe all kinds of things that are (to estimate) hundreds of times crazier and weirder — for example, that the equations describing how electrons behave are different depending on whether somebody is looking or not, that there is literally no such thing as the microscopic world, or there is but it is scientifically impossible to provide a direct and literal description of it in ordinary physical-scientific terms. Or even take the analog/extended action-reaction principle specifically: is this respected when a measurement interrupts the unitary evolution of some system’s quantum state and makes it instead collapse? To even begin to answer that, you’d presumably have to give a much clearer account of the ontology and dynamics of ordinary QM than anyone has ever bothered to give. That is, the answer to the question is buried under all the vague handwaving talk that constitutes the fundamental problem with ordinary QM in the first place. That is, the only reason you can get away with making a criticism like this action-reaction business against the pilot-wave theory, is that the orthodox version of QM is such a stinking mess that you don’t even recognize that you can’t even begin to question whether it respects, or not, this supposedly important principle. That is, the pilot-wave theory is here being criticized, unfairly, precisely for its main virtue — that it sweeps away the stinking mess of vague, inconsistent talk and presents something so clear and precisely-formulated that it becomes possible to raise silly, reaching, nit-picky objections and thereby lose sight of its clear overall superiority to the orthodox theory.James Swingland“…in my old copy of Quantum Mechanics by Rae, there is an important critique (pg 261). Take a hydrogen atom. The electron (in the de Broglie-Bohm picture) has a definite location. this means the atom should have an electric dipole moment. If there is no dipole moment, the charge must be associated with the pilot wave, in which case - what reality does the particle have? Similar arguments are found in Advanced Quantum Mechanics: Materials and Photons by Dick, who I think strengthens the case slightly. The same arguments can theoretically be applied to gravity as well. In the absence of positive experimental evidence, this counts strongly against simple versions of the pilot wave.”Ordinary QM also says that the atom should have an electric dipole moment (if we look). I guess the objection is supposed to be that the pilot-wave theory says it will have one even when we don’t look, whereas ordinary QM says it will not have one when we don’t look. So the two theories make different predictions for what we will see when we don’t look?That’s a bit tongue-in-cheek, obviously, but come on people. The pilot-wave theory makes the same empirical predictions as ordinary QM. So you’re not going to succeed at this “gotcha” kind of thing — finding a situation where it just flatly makes the wrong prediction. Here I suspect the actual error is in assuming that, since the pilot-wave theory provides (just like classical mechanics!) a consistent and comprehensible story about what is going on microscopically, it must actually just be classical mechanics. That is, it must say that the way a Hydrogen atom interacts with other things in its environment, is exactly like how classical mechanics says a pair of nearby oppositely-charged particles (a “classical Hydrogen atom”) would interact with stuff in their environment. But the pilot-wave theory is not classical mechanics and doesn’t say that. If you want to know what the theory says will happen if you put a Hydrogen atom near another Hydrogen atom, or near a functioning Hydrogen-atom-dipole-moment-measuring-device, or whatever, then take the time to figure out what the theory actually says. Spoiler alert: it says the same thing that you would have said if you (correctly) analyzed the same situation using ordinary QM.Ron Maimon“One reason to be sure that pilot-wave is not even a reasonable formulation of quantum theory as it is usually stated is that the position basis is not particulary distinguished in quantum mechanics, but it is distinguished in pilot-wave theory. Pilot wave has particles with definite positions, not definite momenta, which wander around guided by the wave (over positions).”The particles in pilot-wave theory do have definite momenta. I mean, they have definite positions, at all times, and hence at every moment there is the instantaneous rate of change of position we usually call “velocity” and you can multiply that by the mass and call it the momentum. As I mentioned above, what’s true is that position measurements reveal the pre-existing position, whereas momentum measurements do not (in general) reveal the pre-existing momentum. (A simple example here is a 1D particle-in-a-box in the ground state. As it turns out, the pilot-wave theory says that the (literal pointlike) particle being guided by this particular wave function will be at rest, like the electron in a H atom in the ground state. So its actual momentum is zero. But if you measure its momentum you will get either approximately +hk or approximately -hk, where “h” is h-bar, and “k” is pi/L. This is not so mysterious. The act of measuring the momentum makes the wave function change, which makes the particle start moving. For example, one might “measure the momentum” by removing the walls of the box, letting the particle evolve freely for an hour, and then measuring its position. But as soon as you remove the walls, the wave function will develop from the stationary ground state into two oppositely-propagating wave packets…).Anyway, again, the theory is often criticized for supposedly breaking some sacrosanct symmetry between position and momentum representations. But this criticism is silly. There is no such symmetry in the first place. And the pilot-wave theory doesn’t break it in anything like the way the critics always claim it does.“You could repeat Bohm's trick in the momentum basis, and get particles with definite mometum wandering around in the space of all their simultaneous momentum (the formulation is a bit more difficult because the force laws are not always quadratic, but ignore this, you can do it in principle). You can do it for any basis. So there are an infinite number of different Bohm theories which only differ in which variable you choose to make "real", all these formulations give the same predictions at the end of the day. This is an uncomfortable position, since there can be no test to determine which one is correct. It also means that the formulation has made an unnecessary arbitrary choice of a distinguished basis, and so is not very nice theoretically.”Such alternative versions/theories would absolutely not “give the same predictions at the end of the day”. Indeed, such versions/theories wouldn’t even predict a world with the right sort of gross macroscopic features, such as stable tree- and table-shaped collections of particles. (Note that it is important here to remember that the outcomes of experiments are to be found, in the pilot-wave theory, in the particle configurations. If you just ignore the particles, and assume that the outcomes are to be extracted from the wave function, using some ad hoc rules, like in ordinary QM, then obviously the particles can be doing anything you want and it won’t affect anything. But that’s not a serious criticism; it amounts to “if I ignore the whole reason this theory exists, the theory seems to have no reason to exist”.)There is a similar kind of freedom in the theory, though, against which an objection of the same general spirit could be made. This is a bit technical, but there is a whole family of guidance equations which all give rise to the same quantum statistics. People usually just take the simplest one (and sometimes people argue about which one is simplest, or how best to decide which one is simplest) but in principle you could insist that “This is an uncomfortable position, since there can be no test to determine which one is correct” and hence one must make “an unnecessary arbitrary choice… so it is not very nice theoretically.” This is another one of the sorts of things that I think could make for an interesting discussion and/or research program, but is ridiculous as an answer to the question that began this thread. Anybody who actually cares about things being “very nice theoretically” will despise orthodox QM with the rage and fire of a thousand highly active volcanoes. So the idea that we could explain why most physicists prefer orthodox QM to the pilot-wave theory, by reference to their finding some tiny quibbling detail like this about the pilot-wave theory “not very nice theoretically”, is just laughably absurd.Ian Miller“For me, there are problems with the pilot wave theory, which I have tried to rectify in my Guidance Wave interpretation. The biggest problem is, if the pilot wave is to direct the particle’s motion, then surely the phase velocity should equal the particle velocity? To do that, then the phase velocity should equal E/p, in which case E has to be twice the kinetic energy. So, where is that extra energy? That is my hidden variable.”In the actual pilot-wave theory, the velocity of the (literal pointlike) particle corresponds to the group (not phase) velocity of the associated wave. (That is basically what the guidance equation says.) Note that this automatically and immediately implies something rather nice — namely, that if the wave function is a propagating wave packet, the particle will more or less stay with it as it propagates rather than racing way ahead or falling way behind.I fully support explorations of new ideas, but it sounds like in this case your alternative interpretation may have been motivated by a simple mistake.Michael Price“In Bohm's pilot wave theory the wavefunction does not collapse. Hence Bohm's theory implies many worlds, and the postulated "hidden variable" particles are superfluous. By Ockham's razor the particles can be discarded. It is as David Deutsch said: "pilot wave theories are just many worlds in denial."”The conclusion, that “Bohm’s theory implies many worlds”, only follows from “the wavefunction does not collapse”, if one assumes that worlds are made out of wavefunction. This is indeed one of the fundamental assumptions of Everett’s many worlds theory, but it is not an assumption that is shared by the pilot-wave theory. So this argument doesn’t really work in the way intended by Deutsch.To be neutral about it, I think one could agree on the following: the ontology of the Everett theory (that is, the universal wave function) is completely shared by the pilot-wave theory; the pilot-wave theory just adds something additional, namely, definite evolving positions for N (literal pointlike) particles. And so, to whatever extent the universal wave function can really be understood as a description of some huge number of parallel, non-interacting worlds (of some basically-familiar sort involving, for example, matter of some kind that is distributed somehow throughout ordinary 3-space) the two theories both evidently include all of those worlds — and then the pilot-wave theory includes one additional one, the one made instead of particles.Everettians essentially just take for granted that the wave function can be understood in this way, and they do this despite the serious (and to my mind intractable) problems associated with the fact that the wave function is, at best, a field on an abstract high-dimensional configuration space. It is simply not obvious how this mathematical object could describe anything like what the Everettians assume it describes. People who prefer the pilot-wave theory tend to say that the problem with the many worlds interpretation is not that it has too many worlds, but that it has none at all. The beauty of the pilot-wave theory is that no baseless assumptions and no handwavy philosophical speeches are needed to see how a world of the appropriate sort emerges from the basic postulates of the theory: one has N particles moving around in 3-space, so one immediately (by coarse-graining) has the kind of matter distribution that we need to correspond to what we see around us. The problem, for the pilot-wave theory, associated with the wave function is not that this implies some embarrassing plethora of additional, unwanted worlds; the problem instead is how to understand the wave function in physical terms at all. Is it a field? In configuration space? What does that even mean? Is it instead some kind of abstract, indirect description of some kind of stuff (fields or particles or ???) in ordinary 3D physical space? If so, what kind of stuff, and how does the description work, and how could we reformulate things to talk about this other stuff in a more direct way? Or is the wave function not really a physical thing (or an indirect description of physical things) at all, but instead something more like a law? Or what?Eric Pepke“Because nobody seriously cares. Quantum interpretations are for philosophical late-night discussions over beer (experimental physicists) or coffee (theoretical physicists) or when you want to shut your brain up to go to sleep.”Actually lots of people do care, and more (serious, smart, highly-educated, scientific) people would care more if they understood the issues better, instead of being deluded by misconceptions and misinformation and invalid arguments. Maybe this long post will help a tiny bit. One thing is for sure: having just written this in one sitting, I desperately need both coffee and beer.
What was it like to be in Prof. Amar Bose's class at MIT?
I took Dr. Bose's Acoustics course (6.312) around 1994. Professor Bose was one of my favorite lecturers at MIT, hands down. He cared about teaching, he cared about the students, and he cared about the class.First off, you can't ignore the name recognition. "Bose... as in THE Bose?" You think he knows something about acoustics? Yup.Secondly, there were the lectures themselves. Dr. Bose was very clear with his equations. He would punctuate discussions with stories: some personal, some academic, some business. (Long time TAs knew all of his stories by heart but still loved hearing them.) He would often circle back around at the end of his lectures to make a point that he foreshadowed at the beginning -- so it was a complete story rather than a run-on firehose of information. He had a very well planned out syllabus. Many of his lectures have been preserved: see Amar G. Bose: 6.312 (Acoustics) | Teaching Excellence at MITThirdly, there were the real-life applications of his theory. One problem set involved buying an Orangina bottle from the store and calculating its resonance based on its shape, to guess what approximate musical note it should play when you blow on the top. Another long class derivation eventually came down to this 2:1 ratio in the length of the wave guide tubes, which, as Dr. Bose told us, was much easier to patent than complicated wave equations. All of his speakers have that 2:1 ratio in them, and he just proved why it was mathematically superior for efficiency in output. He even took us all on a plant tour at the end of the class, to show equations transformed into production.Fourth, his Teaching Assistants (TA's) were incredibly helpful, at least the year I took the course. They had long office hours available for coaching. They clearly knew the material. And they were passionate about student success. I'm pretty sure he personally knew and picked them -- further evidence of how much he cared about the learning process.And finally, there were the tests, which to this day remain the epitome of how I think college classes should assess their students' learning.a) The three tests were each 2 hours long, "but we T.A.'s will stay as long as you want until you're satisfied with your answers." (Tests began at 7pm and TAs sometimes stayed till midnight.)b) The tests were open book: bring all your notes, textbooks, basically anything but a phone to contact a friend.c) The tests were 5 questions. The first question was 10 pts and could basically be copied out of lecture notes -- if you attended class, you got it. The remaining 4 questions and 90 pts were entirely brand new problems made up just for that year's test (so "course bibles" weren't helpful). They required combining principles you learned in class in interesting and unique ways to solve a complex problem or explain a reported observation. For instance, I vaguely remembered one that talked about how it was easier to hear people talking from across a certain lake when the weather was colder in the morning than at other times. It required understanding the theory first, then applying some thermo/temperature calculations and making some reasonable assumptions about how the sound waves would act traveling over the water.d) About an hour into each test, the TAs at the front of the room stood up and asked for our attention. They explained that Dr. Bose had bought ice cream for everyone. We put our pencils down and came up to the front, where several pints of a variety of Toscanini's ice cream were available. With that sugar rush in hand, we returned to our desks, spoon and dish in hand, and continued with the test. The first time was a surprise; the next two times it was a test-taking strategy to plan around. ("Okay, I'll finish problem #3 before the ice cream, and then after the short break I'll be ready to come back to #2.")I got a solid B in the class... but it inspired me to pursue speech recognition as a concentration for my studies, which eventually led to the start of my career (at Dragon Systems, working on Dragon NaturallySpeaking) and tied together my music minor with my electrical engineering major perfectly.
Does Bohmian mechanics explain entanglement?
Question originally answered : Does Bohmian mechanics explain entanglement?Well, as I understand it, Bohmian mechanics might well provide an explanation for entanglement. Whether or not it is the explanation is for the philosophers to debate.The basic idea of Bohmian mechanics is that there exists some substance permeating the entire universe in which there exist waves. It is these wave that interact with particles moving through space ‘guiding’ the actual path that particles take, hence the name ‘guiding wave’.It is also assumed that particles actually at all times actually have definite positions, something not assumed in standard interpretations of QM.This interaction between particles and the guiding wave is a two way street. That is to say that the guiding wave influences the path of the particles and, in turn, the particle influences the precise form of the guiding wave.Note that there would then be two—not one—wave equations, present. We have the standard Schrödinger’s equation, and a new equation describing the guiding wave.The end result of this mechanism would be a system that is inherently non-local, in the sense that the guiding wave is fully dependent on what each and every particle has ever done. Perhaps a simple analogy might help understand.Imagine the guiding wave as the ripples in a fluid. Imagine a particle as little ball, bouncing around on top of that fluid. The wave will be pushing the particle in certain directions every time the particle bounces on its surface, and reciprocally, the particle will cause little ripples in the fluid that spread out and change the guiding wave. So at any time, the exact shape of the wave is determined by the complete history of every single particle that has ever bounced on the fluid. At least that would be the case if the fluid were internally frictionless and the energy in the waves did not dissipate. The consequences of each bounce would live on forever in that case. This would in principle be a fully deterministic model, in the sense that, given the initial conditions (starting guiding wave shape and the initial disposition of all particles) any future state could be calculated by a deterministic algorithm to any desired degree of accuracy.Now, to answer your question. This might form the basis of an explanation for entanglement, because the interaction between the particles that caused them to become entangled, would leave a permanent marker—a subtle change—on the guiding wave. This would already hold true for each individual particle. Because of this, even though the entangled particles may become widely separated spatially, each particle would nonetheless always feel both the effects caused by itself to the guiding wave (its own history), but also the effects of all other particles, in particular that would include the ‘entangled’ partner particle.The correlation established by the entanglement would in essence be permanently recorded in the dynamic structure of the guiding wave. But personally, I feel that this would imply that, basically, all particles are entangled with all other particles at all times, essentially making the very concept of entanglement vacuous. The entire histories of all particles would influence the future of all particles, including themselves. Any interaction anywhere would always be correlated with all other interactions, however subtly.I have not studied Bohmian mechanics in any real detail, so I am definitely not sure that that last remark holds water, but I present it anyway as the feeling I left the topic with.Now, as to whether or not that actually could be an explanation, there has been some work done studying physical systems following that model. Perhaps Fluid Tests Hint at Concrete Quantum Reality might be an interesting read.In that article it is mentioned that models of this type can actually manifest phenomena very reminiscent of quantum phenomena. There are phenomena resembling quantum tunneling and discrete energy levels in bound states. From that link the following:The quantum statistics are apparent even when the droplets are subjected to external forces. In one recent test, Couder and his colleagues placed a magnet at the center of their oil bath and observed a magnetic ferrofluid droplet. Like an electron occupying fixed energy levels around a nucleus, the bouncing droplet adopted a discrete set of stable orbits around the magnet, each characterized by a set energy level and angular momentum. The “quantization” of these properties into discrete packets is usually understood as a defining feature of the quantum realm.The double slit experiment (quite possibly part of the original motivation for proposing guiding waves) can be done with similar results, etc.It does make for an interesting read. But according to that same article:In its current, immature state, the pilot-wave formulation of quantum mechanics only describes simple interactions between matter and electromagnetic fields, according to David Wallace, a philosopher of physics at the University of Oxford in England, and cannot even capture the physics of an ordinary light bulb. “It is not by itself capable of representing very much physics,” Wallace said. “In my own view, this is the most severe problem for the theory, though, to be fair, it remains an active research area.”
- Home >
- Catalog >
- Legal >
- Work Statement Template >
- Artist Statement Examples >
- Artist Statement Example >
- photography artist statement examples >
- Name: The Wave Complete Study Guide