A Premium Guide to Editing The Chemistry 111 - Laboratory Report Form Experiment 2
Below you can get an idea about how to edit and complete a Chemistry 111 - Laboratory Report Form Experiment 2 in seconds. Get started now.
- Push the“Get Form” Button below . Here you would be introduced into a webpage that enables you to carry out edits on the document.
- Select a tool you need from the toolbar that appears in the dashboard.
- After editing, double check and press the button Download.
- Don't hesistate to contact us via [email protected] for additional assistance.
The Most Powerful Tool to Edit and Complete The Chemistry 111 - Laboratory Report Form Experiment 2
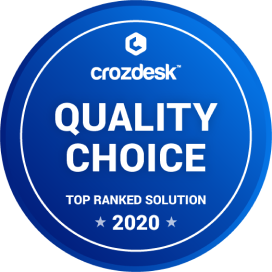
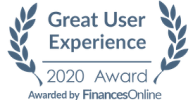
A Simple Manual to Edit Chemistry 111 - Laboratory Report Form Experiment 2 Online
Are you seeking to edit forms online? CocoDoc has got you covered with its detailed PDF toolset. You can get it simply by opening any web brower. The whole process is easy and beginner-friendly. Check below to find out
- go to the CocoDoc's free online PDF editing page.
- Import a document you want to edit by clicking Choose File or simply dragging or dropping.
- Conduct the desired edits on your document with the toolbar on the top of the dashboard.
- Download the file once it is finalized .
Steps in Editing Chemistry 111 - Laboratory Report Form Experiment 2 on Windows
It's to find a default application which is able to help conduct edits to a PDF document. Luckily CocoDoc has come to your rescue. Examine the Instructions below to know possible methods to edit PDF on your Windows system.
- Begin by acquiring CocoDoc application into your PC.
- Import your PDF in the dashboard and make alterations on it with the toolbar listed above
- After double checking, download or save the document.
- There area also many other methods to edit PDF text, you can get it here
A Premium Manual in Editing a Chemistry 111 - Laboratory Report Form Experiment 2 on Mac
Thinking about how to edit PDF documents with your Mac? CocoDoc has come to your help.. It enables you to edit documents in multiple ways. Get started now
- Install CocoDoc onto your Mac device or go to the CocoDoc website with a Mac browser. Select PDF file from your Mac device. You can do so by hitting the tab Choose File, or by dropping or dragging. Edit the PDF document in the new dashboard which encampasses a full set of PDF tools. Save the content by downloading.
A Complete Guide in Editing Chemistry 111 - Laboratory Report Form Experiment 2 on G Suite
Intergating G Suite with PDF services is marvellous progess in technology, a blessing for you cut your PDF editing process, making it quicker and more time-saving. Make use of CocoDoc's G Suite integration now.
Editing PDF on G Suite is as easy as it can be
- Visit Google WorkPlace Marketplace and search for CocoDoc
- establish the CocoDoc add-on into your Google account. Now you are more than ready to edit documents.
- Select a file desired by clicking the tab Choose File and start editing.
- After making all necessary edits, download it into your device.
PDF Editor FAQ
What is the syllabus of m.SC environmental science in JNU?
I could not understand if you are looking for syllabus of M.Sc in Environmental Course for your coursework or for preparation of entrance exam. If you are looking for coursework in Jawaharlal Nehru University, New Delhi; here you go.The M. Sc. programme is spread over four semesters. It carries 64 credits and comprises of four different components viz: I) Teaching II) Lab Work III) Field Work and IV) Dissertation.Distribution of credits for M. Sc. Programme is:Total Credits for M. Sc. Degree Programme = 64 credits I) Teaching = 50 creditsII) Lab work, Field work and Dissertation = 14 creditsDistribution of credits for teaching (Total 50 credits)i) Core courses = 26 credits ii) Optional courses = 24 creditsDistribution of credits for Lab work, Field work and Dissertation (Total 14 credits)a) Lab work = 6 credits (Lab Work I =3 credits; Lab Work II =3 credits)b) Field work = 3 creditsc) Dissertation = 5 creditsI) Teaching (50 credits)Teaching is a major component of the programme. It shares 50 credits out of total 64. The remaining three components i.e. Lab work, field work and dissertation share remaining 14 creditsVarious courses offered under M. Sc. programme are categorized as:A) Core courses B) Non Credit courses and C) Optional courses.Altogether there are 46 courses: 13 as core, 2 non credit courses and 31 optional courses. All core courses are offered in I and II semesters and all optional courses are offered in III and IV semester of the M. Sc programme.All Core Courses are of 2 credits each and compulsory for all the students. Non credit courses do not carry any credits, however, as per the JNU ordinance, completion of such courses by every student is a mandatory requirement for the award of the degree. Optional courses are of 3 credits each and cover all specialized courses across different sub disciplines of environmental sciences namely; Mathematics, Physics, Statistics, Geology, Chemistry and Biology. There is a running list of 31 optional courses, out of which students will have to choose any 8 optional courses (four in each semester) to obtain 24 credits.II) Lab work, Field work and Dissertation (14 credits)a) Lab work (6 credits)The lab work component is spread over first two semesters and is called as Lab work I and Lab work II to be completed in I and II semesters respectively. Under Lab Work I and II, sets of experiments specially designed for M. Sc. students by faculty members of the school are carried out in M. Sc. lab or in the lab of the concerned faculty member during the period of five working days in the afternoon.b) Field work (3 credits)To strengthen the field work component and to have a wider exposure of the field conditions, students will undergo extensive field work which will help them in developing the understanding of different aspects of environmental sciences. Field work is completed in second semester. Each student will submit his/her field work report for evaluation.c) Dissertation (5 credits)Each student will work for M. Sc. Project under the supervision of formally assigned supervisor in the school. Assigning of supervisor will be based on academic interest shown by the student in research specialization of the concerned faculty member followed by the consent given by the faculty member to supervise the project work of that particular student. Student shall complete the process of academic interaction to obtain teachers consent to supervise his/her project work by the end of second semester. The work on research project will start in 3rd semester under the supervision of concerned faculty member in his /her lab and will be completed by 4th semester with writing and submission of dissertation. Dissertation will be evaluated by a 3 member expert committee. Students will have to present their work and defend it in an open viva- voce.LIST OF COURSESA) Core Courses (Compulsory for all)(Total courses 13, Total credits: 13 x 2 =26)Remedial Mathematics ES-101ORRemedial Biology ES-102Environmental Chemistry ES-103Earth processes ES-104Ecology ES-105Statistics ES-106Environmental Pollution ES-107Natural hazards and disaster management ES-108Environmental Impact Assessment ES-109Energy and Environment ES-110Remote sensing and Geoinformatics ES-111Environmental Biochemistry and Toxicology ES-112Marine environment ES 113Soil Science ES-114B) Non- Credit Courses (Compulsory for all)Current Environmental Issues ES-11516. Scientific Writings and Ethics ES-116C) Optional Courses – (Total courses- 31 of 3 credits each; Students will have tochoose any 8 courses to obtain total 24 credits)1. Environmental Modeling ES-2012. Climatology ES-2023. Meteorology ES-2034. Noise Pollution ES-2045. Environmental Physics ES-2056. Environmental instrumentation and techniques ES-2067. Geochemistry ES-2078. Groundwater Hydrology ES-2089. Oceanography ES-20910. Natural resource Management ES-21011. Glaciology ES-21112. Biogeochemistry ES-21213. Environmental Geology ES-21314. Water Resources ES-21415. Air Pollution Chemistry ES-21516. Water Pollution Chemistry ES-21617. Soil Pollution Chemistry ES-21718. Solid and Hazardous Wastes Management ES-21819. Metrology ES-21920. Pollution Biology ES-22021. Biodiversity and conservation ES-22122. Forest ecology ES-22223. Microbial Ecology ES-22324. Ecosystem Dynamics ES-22425. Environmental Biophysics ES-22526. Ecology and sustainable development ES-22627. Environmental Xenobiotics and human health ES-22728. Fundamentals of Molecular Biology and Biotechnology ES-22829. Applied biotechnology and Bioremediation ES-22930. Eco-toxicology ES-23031. Environmental and Occupational health ES-231D) Lab Work1. Lab work I (3 credits) ES-2322. Lab work II (3 credits) ES-233E) Field Work (3 credits) ES-234F) Project Work (5 credits) ES-235-----------------------------------------------------------------------------------COURSE CONTENTA) Core CoursesRemedial Mathematics ES-101 (for Non- Mathematics students)Functions- polynomial, logarithmic, exponential, absolute value, trigonometric. Limits, Indeterminate forms, Continuity. Derivability. Differentiation of simple mathematical functions- product rule, quotient rule and chain rule. Integration- by parts, substitution and by partial fractions. Linear differential equations and their solution. Introduction to Matrices and Determinants. Introduction to Vectors- addition, subtraction, multiplication of vectors. Equation of Straight Line and Solving Linear System of Equations.OR2. Remedial Biology ES-102 (for Non- Biology students)History and scope of ecology, Evolution of biosphere, Diversity of life forms. Biological communities, species interaction, Communities properties, succession. Plant diversity and nomenclature with major classes of plants; Phytogeographical regions; Rare and threatened plants and exploration of plant wealth. Animal diversity and categories of animals; Rare and threatened species of mammals, aves, reptiles, pisces etc.; Exploration and conservation of faunal wealth. Microbial diversity, bacteria, fungi, actinomycetes; Microbial diversity in man-made ecosystems and natural ecosystems. Importance of flora and fauna in nutrient cycling, its effect, degradation and metabolism.3. Environmental chemistry ES-103Fundamental Chemistry: Elements, Chemical bonding, chemical reactions and equations, Organic functional groups, classes of organic compounds. Free radical reactions, catalytic processes.Elemental cycles (C, N, S, O) and their environmental significance.Fossil fuels: their types, properties, combustion and environmental implications.Atmospheric constituents, Green house gases and climatic changes. Chlorofluorocarbons and their substitutes. Photochemical smog. Water quality and wastewater treatment. Role of soaps, detergents and phosphorus fertilizers in eutrophication. Persistent organic pollutants: pesticides usage, toxicity and their environmental degradation. Earth crust and weathering mechanism; Soil formation and chemical characteristics. Chemical classes of Hazardous waste, their effects on the environment. Chemical treatment of hazardous wastes.4. Earth Processes ES-104Evolution of various branches of Geology. Origin of the earth. Primary differentiation and formation of core, mantle, crust, atmosphere and hydrosphere. Magma generation and formation of igneous and metamorphic rocks. Concept of Minerals and Rocks. Weathering, erosion, transportation and deposition of earth’s materials by running water, wind and glaciers. Formation of land forms and sedimentary rocks. Plate tectonics- sea floor spreading, mountain building, evolution of continents and structural deformation. Thermal, magnetic and gravitational fields of the earth. Concepts of engineering and urban geology.5. Ecology ES-105History and scope of ecology, autecoloty, synecology, population, community, biome, tolerance range and limiting factors. Distinguishing characters of forests grasslands, arid lands and wetlands; community organization- concept of habitat, functional role and niche, key stone species, dominant species, ecotone, edge effect. Analytical characters, synthetic characters like forms, species diversity and measurement of diversity. Population dynamics, models for single and interacting population, stable points, stable cycles, chaos competition, prey predation, etc. Ecological succession, primary and secondary processes in successions, models of successions, climax community and types of climax. Vegetation of India. Fundamentals of Microbial ecology. Microbial metabolism and microbial interaction. Biochemistry of biological nitrogen fixation and other microbial Pathways in terms of enzymology.6. Statistics ES-106Measures of central tendency. Measures of dispersion. Measures of skewness and kurtosis. Probability- definition, addition and multiplication laws,concept of random variable. Probability distributions- binomial, poisson and normal. Sampling theory- hypothesis testing and interval estimation for large samples. Chi-square test, t-test and F-test of significance. Correlation and regression. analysis. One way analysis of variance.7. Environmental Pollution ES-107Linkage between energy, environment and development. Human population issues. Definition of pollution. Different types of pollution- Air, Water and soil and their local, regional and global aspects. Air: Sources of air pollutants, their behavior in the atmosphere. Effects of air pollutants on humans, animals, plants and properties. Control approaches. Water: Sources, effects, water pollution treatment. Soil: Sources and nature of soil pollution and its harmful effects. Solid waste: generation, collection, environmental effects and safe disposal practices. Environmental problems associated with noise pollution, oil pollution and radioactive pollution.8. Natural hazards and disaster management ES-108Introduction to Hazards- Hazard classification-types of hazards ;Natural Hazards: causes, (continental drift, plate tectonics, sea floor spreading, isostacy, etc.,) distribution pattern, consequences and mitigation: Earthquake, Tsunami, Volcanoes, Cyclone, Flood, Drought, Landslide, cold and heat hazards, forest fire, etc.,- causes, types, distribution adverse effects, etc.,- Disaster introduction- disaster Management Capability-Vulnerability- risk- preparedness and mitigation- Disaster management cycle- community planning education and Engineered structure /structural strengthening techniques- Hazard zonation and mapping- Risk Reduction Measures.9. Environmental Impact Assessment ES-109Linkage between development and environment; global commons: carrying capacity: origin and development of EIA: relationship of EIA to sustainable development: EIA in project planning and implementation: EIA process: evaluation of proposed actions, scoping and base line study, identification and prediction of impacts, mitigation measures. Comparison of alternatives, review and decision making, public participation and compensatory actions: green belts: National Environmental Policies and guidelines in India. Conditions and approach for EIS review. Case studies: river valley projects: thermal power plants: mining projects: oil refineries and petrochemicals.10. Energy and Environment ES-110Energy resources and their exploitation, Sun as source of energy- nature of its radiation, Conventional energy sources: coal, oil, biomass and nature gas, non-conventional energy sources: hydroelectric power, tidal, wind, geothermal energy, solar collectors, photovoltaics, solar ponds, nuclear-fission and fusion, magneto-hydrodynamic power (MHD), Energy use pattern in different parts of the world and its impact on the environment. CO2 emission in atmosphere. Mechanism of radiation action on living systems- Stochastic and Non-stochastic effects; delayed effects, radioactivity from nuclear reactors, fuel processing and radioactive waste, hazards related to power plants, terrestrial and non terrestrial radiation, dose from environment and nuclear radiations, ultraviolet radiations, pathways analysis and dose assessment, radiologic age dating, radioactivity risk assessment, criterion for safe exposure.11. Remote sensing and Geo- informatics ES-111Introduction to Remote sensing & GIS. Principles of remote sensing & GIS. Spectra of Environmental Components. Terrestrial and Extra terrestrial satellites in Remote sensing and GIS. Remote sensing & GIS applications on Ocean, Atmosphere, Land, Geology, Water Resources (Ground water and Surface water). Cryosphere, Disaster, Defence studies. Use of softwares in Remote sensing and GIS to solve Environmental problems including Groundwater Exploration, Rainwater Harvesting, Biomass analysis and its relationship with Georesource evaluation. Use of Remote sensing and GIS in development of Early warning system to monitor Agriculture. Identification of Genetically modified crops in correlation with water quality and soil moisture by using Remote sensing & GIS. Applications of Remote sensing and GIS in early warning of Tsunami, Earthquake, Snowfall, Global warming, Forest fire, Landslide, Landsubsidance. Use of LANDSAT, SPOT, IRS ERS, RADARSAT and Extra terrestrial satellite data by using ERDAS, ARCGIS, ERMAPPER, IDRISI ENVI and S+ software for solving the Environmental problems. Sun-earth cosmic connection to understand environment of the Earth.12. Environmental Biochemistry and Toxicology ES-112Environmental physiology with considerations of intermediary metabolism- approaches for studying energy metabolism and body temperature changes; Thermo regulation and adaptation. Oxygen uptake from the environment, respiration and metabolism. Electron transport system and oxidative phosphorylation. Photosynthesis: C1, C3, C4 pathways and their regulation. Photorespiration. Biochemistry of altered membrane permeability, free radical formation, lipid peroxidation, lysosomal degradation, superoxide dismutase. Environmental pollutants and their effects on living system. Biochemical approaches to the detoxification of xenobiotics through cellular metabolism.13. Marine Environment ES-113Introduction-Classification- open ocean- shallow marine and deep sea environment- marine resources- marine ecology- marine organisms-productivity- coastal environment-coastal water movement- beaches- coastal dunes- barrier islands- cliffed coast- deltas-coast line- estuaries-mangroves- lagoons- salt marshes- coral reefs- classification of marine sediments- clay minerals- biogenic silica- evaporites- nutrient in oceans- carbon and global climate change- marine pollution- law of the sea.14. Soil Science ES-114Soil forming rocks and minerals- Classification- Weathering of rocks and minerals- Processes of weathering and factors affecting them. Soil formation- Factors of soil formation- Soil forming processes- Profile development- Definition of soil- Soil composition. Soil physical properties- Soil separates and particle size distribution- Soil texture and structure- Bulk density, particle density, pore space, soil air, soil temperature, soil water, soil consistence - Significance of physical properties to plant growth. Soil chemical properties- Soil colloids- Inorganic colloids- Clay minerals- amorphous- Ion exchange reactions- Organic colloids- Soil organic matter- Decomposition- Humus formation- Significance on soil fertility, Soil reaction- Biological properties of soil- nutrient availability.B) Non Credit Courses (Compulsory for all )1. Current Environmental Issues ES-115Contemporary and emerging environmental issues of local, regional and global significance. Broadly the topics will be pertaining to: i) Linkage between population, development and environment ii) climate change ii) stratospheric Ozone depletion iii) water resources iv) environmental toxicants and human health v) biodiversity conservation and vi) environmental episodic events, etc.2. Scientific Writings and Ethics ES-116Overview of Moral and Ethical questions in Scientific writing. Overall outline and structure of the article/manuscript. Description, value, and development of points/outlines before writing. Screening of Material for inclusion within the structure of the manuscript.Importance of Authors and their sequence, importance of clear title, abstract or summary. Introduction, Methods, Results, and Discussion. Numbers and statistics, Tables and Figures, Discussion. Writing Style: Active or passive, Punctuation, use of commas, apostrophe, semicolon and colon. Avoiding duplication and repetition. Importance of revisions and references.Plagiarism, paraphrasing and copy write violation. Consequences of plagiarism. Why not to fudge, tinker, fabricate or falsify data. Examples.C) Optional Courses1. Environmental Modeling ES - 201Role of Modeling in Environmental Science. Model Classification- Deterministic Models, Stochastic Models, Dynamic Models, Steady State Models. General Steps Involved in Modeling, Mass Balancing, Energy Balancing, Microbial Growth Kinetics- Exponential Growth Model, Logistic Growth Model, Monod Equation, Two Species Population Growth Model of Competition. Lotka-Volterra Prey-Predator Model, Oxygen Sag Model, Gaussian Plume Model.2. Climatology ES - 202Elements of climate, climate controls, Earth's radiation balance, latitudinal and seasonal variation of insolation, temperature, pressure, wind belts, humidity, cloud formation and precipitation, water balance, spatial and temporal patterns of climate parameters, Air masses and fronts, SW and NE monsoon, jet stream, tropical and extratropical cyclone, ENSO, QBO. Classification of climate- Koppen's and Thornthwaite' scheme. Climate change3. Meteorology ES - 203Meteorology fundamentals- Thermal structure of the atmosphere and its composition, Pressure, temperature, wind, humidity, moisture variables, virtual temperature, radiation, radiation from sun, solar constant, surface and planetary albedo, emission and absorption of terrestrial radiation, radiation windows, greenhouse effect, net radiation budget, atmospheric stability diagrams, turbulence, diffusion, dry and moist air parcel, thermodynamic diagrams, T-phigram and mixing height, thermodynamics of dry and moist air, specific gas constant, adiabatic and isoentropic processes, entropy and enthalpy, adiabatic processes of moist air4. Noise Pollution ES - 204Basic properties of sound waves, sound propagation, Definition of Noise, Health Effects of Noise, Concept of sound pressure level (SPL), decibel scale, addition of decibels, Frequency Response of Human Ear, Equal Loudness Contours, Weighting Networks, Octave Bands, Measurement and analysis of sound. Percentile Indices of Noise, Equivalent sound pressure level (Leq), Noise pollution level (NPL), Sound exposure level (SEL), Traffic noise index (TNI), Day-Night level (DNL), noise criteria curves; Noise sources; Industrial Noise and Traffic Noise, Noise control and abatement measures; absorbing materials, barrier materials and damping materials. Acoustic silencers and mufflers.5. Environmental Physics ES - 205Concept and scope of environmental Physics with respect to human environment; built environment; urban environment; global environment. Laws of thermodynamics, irreversible thermodynamics and entropy. Wind chill, Hypothermia. Heat balance (steady and transient), Electromagnetic Radiation, Thermal regulation in buildings- Thermal insulation, Thermal conduction effects, Convection effects, Radiation effects, U-values, Energy use and efficiency in buildings. Energy losses, calculation of energy losses, energy gains.Air regulation in buildings, heat pumps, condensation. Buildings of the future. Nano materials: their properties and influence on human health, environment, communication sector and energy. Method of preparation and Applications of nano materials.6.Environmental Instrumentation and Techniques ES - 206Physics of Dielectrophoresis and its environmental applications, Basics of NMR instrumentations, significance of relaxation time, Raman effect and experimental measurement, Raman Spectroscopy, LASER based techniques, LIDAR based methods and techniques, SODAR Radiofrequency measurement and techniques.7. Geochemistry ES - 207Atomic properties of elements, the periodic, table and geochemical classification of elements; abundance of elements in the bulk earth, crust, hydrosphere, atmosphere and biosphere; introduction to mineral structures and compositions; thermodynamic classification of elements into essential, structural, major and trace elements and their partitioning during mineral formation; chemical reactions involving proton and electron transfers, mineral stability diagrams and controls on the chemistry of natural waters; geochemical cycling-concepts with an example; radioactivity, decay of parent and growth of daughter nuclides and methods of radiometric dating; stable isotopes, their fractionation and application to geothermometry and paleoclimates. Interpretation of XRD and XRF data for Environmental components. Geochemical sample preparation. X-Ray Fluorescence. X-Ray Diffraction. Ion Chromatography, AAS and its interpretation.8. Groundwater Hydrology ES - 208Definition and concept of hydrology and hydrogeology. Distribution of water in the earth’s crust. Hydrological cycle. Genetic types of groundwater and residence time of groundwater, Geological control of groundwater, Vertical distribution of groundwater, Types of aquifers, springs and their classification, Classification of rocks with reference to their water bearing properties. Mode of occurrence of groundwater in different geological terrains of India. Darcy’s law and its validity, Determination of hydraulic conductivity, groundwater tracers. Environmental factors on Groundwater level fluctuations and Land subsidence due to changes in subsurface moisture. Effects of excessive use of groundwater resources. Sources of salinity, Chemical analysis of groundwater, Quality criteria for different uses, Groundwater quality in different provinces of India, pollution of groundwater resources. Ghyben-Herzberg relationship between fresh-saline water. Groundwater exploration. Construction and design of different types of wells. Well completion and development. Groundwater development and management: Groundwater development in urban areas and rainwater harvesting, artificial recharge methods. Management of groundwater and groundwater legislation.9.Oceanography ES - 209Introduction- historical, current and future- Earths structure- Physiography of oceans- origin and evolution of ocean basins (Continental and oceanic basins)- Continental drift, sea floor spreading, plate tectonics- shelf and deep sea sedimentation- physical, chemical and biological aspects of sea water- Ocean current (circulation)- Waves properties and motion- tidal currents and characteristics- air-water interface/ exchange, gas solubility and circulation models.10. Natural resource Management ES - 210Definition- land, water, soil, plants and animals: quality of life: renewable and non-renewable resources: Mineral occurrences, prospects: Mineral resources: Mineral reserves, ore minerals, coal, petroleum, oil and natural gas: water- hydropower, including tidal power; ocean surface waves used for wave power, wind- wind power, geothermal heat- geothermal power and radiant energy- solar power: sustainable development, Urban planning Environmental management, Understanding the resource ecology and life-supporting capacity of resources-Economic models: Green building concept- green technology concept.11. Glaciology ES - 211Glacier systems- Structure and morphology of glaciers- Glacial erosion; Landscape evolution under glaciers, glacial landforms- Mass balance- Glacier dynamics, Englacial and subglacial process and fluctuations- Glacier hydrology- Snow and melt water chemistry of- Approaches to Glaciology- Glacier modeling- Glacier and climate change impact- Glaciers- Glacier and water resources- Recent advances in Glaciology- Spatial Data Acquisition Glacier Hazards- Glaciers as tool for palaeo climate studies.12. Biogeochemistry ES - 212Introduction- Biogeochemical provinces- Atmosphere- Lithosphere: weathering process, soil biogeochemistry- Terrestrial systems: photosynthesis respiration- Wetlands: vegetation adaptations- Freshwater and Marine Biogeochemistry: Lakes, ponds, rivers, mangroves, salt marsh and estuaries- Oceans: productivity and limiting nutrient role, carbon chemistry- Global biogeochemical cycles: Nutrient cycles-Advances in biogeochemistry- Sediment biogeochemistry, stable Isotopes in Biogeochemistry and their application to various environmental problems. Nutrient dynamic in the atmosphere, hydrosphere, and Lithosphere. Nutrient budgeting and modeling13. Environmental Geology ES - 213Interior of the earth- minerals and rocks- earth processes- plate tectonics- sea floor spreading, mountain building, rock deformation- evolution of continents and earth quakes, volcanoes, landslides, subsidence, rivers and floods and coastal process- interactions between humans and the geological processes, Environmental Hazards-Pollution of the Environment- Waste Disposal, Natural Resources, and Energy Sources and their exploitation. Past, present and future environmental issues and their affect on the earth and our society.14. Water Resources ES - 214Hydrological cycle- Hydrometeorology and climate- hydrometric networks and catchment morphology- precipitation- evaporation and evapotranspiration- soil moisture-river flow-River, Lakes and Ground water- Occurrence of surface water and groundwater. Movement of water on the surface and below the surface. Springs and Hydrothermal phenomena. Ungauged river basin flow- River bank infiltration and recharge-precipitation analysis- evaporation calculation-river flow analysis- Time variation of stream flow levels- rainfall- runoff relationships- Ecohydrology- urban hydrology- Integrated Water Resource Management (IWRM), Urbanization effect on Water resources. Earthquake, Land subsidence and Water resources. Physical, chemical and biological characteristics of Water resources and water quality data processing and interpretation. Sea water intrusion in aquifer system-structural geological approach. Influence of Sun-Earth cosmic connection on Water resources.15. Air Pollution Chemistry ES - 215Chemical composition of atmosphere, Sources of air pollution. Types of air pollutants, organic and inorganic pollutants, their behavior and fate on local, regional and global scale, monitoring of criteria and non-criteria pollutants. Effects of air pollutants on human health, plants, animals and materials. Pollutants and health effects. Air pollution meteorology: Mixing heights, Wind roses, Inversion conditions, Stability of the atmosphere. Long range transport, plume behavior, Air pollution dispersion. Land-atmosphere-ocean interactions of air pollutants. Photochemistry of troposphere, Inorganic reaction in the atmosphere. Reactions involving organic pollutants, Gas to particle conversion. Ozone depletion, Acid rain, Greenhouse effect, Formation of photochemical smog, CFC, their nomenclature, sources and effect, Atmospheric Brown Cloud. Air pollution control technologies: Concept of clean environment, Green technologies, Carbon sequestration, Chemical methods, Electrostatic precipitators.16. Water Pollution Chemistry ES - 216Physicochemical properties of water, Water use- classifications and water quality standard. Basic principles of contaminant behavior in the environment. Hydrologic cycle. Types and sources of water pollution, Major Water Quality (physicochemical and bacteriological) Parameters and their Applications, Basics of water sampling. Water quality objectives and the major chemical, physical and biological processes necessary for designing and managing modern drinking water and wastewater treatment plants, Principles of coagulation, flocculation, sedimentation, chemical precipitation, porous media filtration, disinfection, ion exchange, adsorption, membrane Processes, advanced oxidation processes, air-stripping and other advanced treatment processes, Major contaminant groups and natural pathways for their removal from water.17. Soil Pollution Chemistry ES - 217Physical Chemistry of Soil: Soil Solution Phase, The Soil/Solution Interface, Surface exchange reactions, Soil acidity, Electrochemistry and the Soil, chemistry of waterlogged soil. Soil Pollution: Inorganic and Organic-Definition of pollution and contamination, sources of soil pollution, Effects of chemical residues on soil, (pesticides, fertilizers, heavy metals etc., Soil salinity and alkalinity, Soil pollution from nitrogen, phosphorus, sulfur, micronutrients or trace elements and radionuclide, land degradation, soil erosion. Soil pollution and climate change: Greenhouse gases production, emission, mitigation, carbon sequestration, soil quality.18. Solid and Hazardous Waste Management ES - 218Solid wastes: Definition, types, sources, characteristics, and impact on environmental health. Waste generation rates. Concepts of waste reduction, recycling and reuse. Collection, segregation and transport of solid wastes Handling and segregation of wastes at source. Collection and storage of municipal solid wastes. Solid waste processing technologies. Mechanical and thermal volume reduction. Biological and chemical techniques for energy and other resource recovery. Composting, Vermicomposting, Incineration of solid wastes. Disposal in landfills: site selection, design, and operation of sanitary landfills; secure landfills and landfill bioreactors; leachate and landfill gas management; landfill closure and post-closure environmental monitoring; landfill remediation.Hazardous wastes: Definition, sources and characteristics: Hazardous waste categorization, generation, collection, transport, treatment and disposal. Legislation on management and handling of municipal solid wastes and hazardous wastes19. Metrology ES - 219Fundamentals of metrology, Chemical metrology, Defining uncertainty of measurements, traceability of standards, validation of method, calibration of method, accuracy and precision of results, selectivity, sensitivity, detection limit, limit of determination, specificity, linearity, analytical error, Accreditation systems, Metrology in environment, QA/QC parameters in environmental studies, use of CRMs (Certified reference materials), inter-laboratory comparison exercise, participation in National and International round Robin tests. Representativeness of sampling site, selection of analytical method, selection of appropriate analytical technique, proper storage of samples with suitable preservative, sample blank, field blank, solvent blank, efficiency of extraction, efficiency of sampling, determination of uncertainty in flow, sample preparation.20. Pollution Biology ES - 220Concepts: Pollutants vs. resources; cycling of materials, tolerance ranges, carrying capacity, bioaccumulation. Air Pollution: Responses of plants and animals, monitoring (e.g. lichens) and control of air pollution by plants. Water pollution: Responses of plants and animals to changes in physico-chemical characteristics; distribution of plants in relation to pollution (microphytes; Phytoplankton, periphyton and moorophytes); Biological monitoring and control of pollution in water. Soil pollution: Responses of plants to soil pollution; changes in soil characteristics by waste disposal, sanitary land fills, mining wastes and human activities, and effects on plants and animals.21. Biodiversity and Conservation ES - 221Biodiversity concepts and patterns, Microbial diversity, Plant diversity, Agrobiodiversity, Soil biodiversity, Economic value of biodiversity, biodiversity losses. Biodiversity hotspots and their characteristic flora and fauna, threatened plants and animals of India, ecosystem people and traditional conservation mechanisms, Biodiversity Convention and Biodiversity Act, IPRs, national and international programmes for biodiversity conservation. Wildlife values and eco-tourism, wildlife distribution in India, problem in wildlife protection, role of WWF, WCU, CITES, TRAFFIC, Wildlife Protection Act 1972. In-situ conservation: sanctuaries, biospheres reserves, national parks, nature reserves, preservation plots. Ex-situ conservation: botanical gardens, zoos, aquaria, homestead garden; herbarium; In-vitro Conservation: germplasm and gene Bank; tissue culture: pollen and spore back, DNA bank.22. Forest Ecology ES - 222Forest and forest environment: Structure of forest ecosystem, major forest types of the world, forest types and forest cover of India, regeneration ecology of forest trees. Forest ecosystem function: Primary productivity of forest ecosystems, litter production and decomposition, nutrient cycling and nutrient conservation strategies, plant water relations. Forest ecosystem management: Forest management systems, joint forest management, forest hydrology, forest fire, application of remote sensing technique in forest ecology, deforestation and sustainable forestry, forest laws, non timber forest products. Role of Biology in management and habitat management techniques. Wildlife farming: Objectives, management design, wildlife products, disease control, breeding. Behavioral, ecology and evaluation.23. Microbial Ecology ES - 223An overview of microbial life and its importance in the environment, Microbial structure and function with special emphasis on Bacteria and Archaea, Evolution and microbial phylogenetic diversity, Microbial nutrition and metabolism with emphasis on microbial metabolic diversity, Environmental factors affecting microbial growth and microbial adaptations to extreme environments (like arctic regions and hot springs), Methods in microbial ecology including introduction to microbial genomics, Microbial habitats (air, soil, subsurface, freshwater, marine and the deep sea), Introduction to geomicrobiology, Natural microbial communities with emphasis on biofilms, Microbial biogeochemical processes of nutrient cycling and biodegradation, Microbial interactions: microbe-microbe interactions, plants as microbial habitats, animals as microbial habitats and human microbiome, Applying microbes in wastewater treatment and solid waste management, Industrial applications of microbes including products for health-pharmaceutical, food and beverage industry and biofuels, Molecular biotechnological applications including genetic engineering for the production of vaccines, diagnostics, biopesticides and transgenic plants, Microbial disease ecology and public health, Transmission of microbial diseases through the environment.24. Ecosystem Dynamics ES - 224The ecosystem concept, abiotic and biotic components. Energy input in ecosystem, standing crop, biomass, primary and secondary production, gross and net production, concept of food chain food web, ten percent law, net community production, methods of measuring productivity, pattern of primary production and biomass in the major ecosystem of the world, Energy flow, Feed back and control. Biogeochemical cycles, gaseous and sedimentary turnover rate and turnover item. Hydrological cycle, carbon cycle, nitrogen cycle, sulphur cycle, phosphorus cycle, nutrient budget, man’s impact on nutrient cycles. Population dynamics.25. Environmental Biophysics ES - 225Cellular function of cell, membrane structure and transport origin and conduction of impulses in nerve cell muscles, methods in bioelectric measurements. Radiation and molecular response, elementary aspects of atomic and molecular excitation, biointeractions with environment, fundamental and applied aspects of extremely low frequency, radio and microwave fields, bioacoustics, biomedical aspects of laser. Magnetic environments and geomagnetic fields, behavioural changes, therapeutic and diagnostic possibilities.26. Ecology and Sustainable Development ES - 226Ecosystem concept in space and time; Ecosystem level processes and landscape level processes; the concept of sustainable development temporal and spatial dimensions; Currencies for evaluations of sustainable development- Biophysical measurements; Environmental degradations and conservation issues; Global change and sustainability issues: Climate change, biological invasion, bio-diversity concerns; Ecosystem and social processes in: (a) Rehabilitation of degraded rural landscape, (b) Rehabilitation of unbalanced soils, (c) Rehabilitation of specialized habitats, e.g. water bodies, mangroves; (d) Mined area rehabilitation participatory research and education environmental decision making with people initiates.27. Environmental Xenobiotics and human health ES - 227Interaction of pollutants with biological systems at different levels, e.g., organism, organs, and cell organelles. Biochemical degradation of pollutants inside the cell as well as cellular interactions with the pollutants. Toxins of plant origin. Stress response in living systems. Toxicogenomics: Human population issues and population genetics. Pharmacogenomics; Epidemiology. Cellular interaction and metabolism of xenobiotics; metabolic disorders. Bioconversion of pollutants: active vs. inactive process; enzymic degradation by monooxygenases; Role of cytochrome P 450 and its multiple forms. Immunology: Immune cell responses, Immunity and Immunodeficiency. Allergy and hypersensitive reactions and disorders of immune responses. Carcinogens and Carcinogenesis. Metal toxicity: chemical form, metal biomacromolecule interaction, teratogenecity.28. Fundamentals of Molecular Biology and Biotechnology ES - 228Basic concepts of molecular biology needed for understanding biotechnology. DNA structure and organization into chromosomes. DNA replication. Repetitive DNA; coding and noncoding sequences in genomes. Gene structure and expression. Mechanics of transcription, translation and their regulation in both prokaryotes and eukaryotes. Key discoveries (restriction enzymes, bacterial plasmids, modifying enzymes) leading to recombinant DNA technology. Overview of basic techniques in genetic engineering: Introduction of cloned genes into new hosts using plasmid and phage vector systems. Expression cloning, affinity purification of expressed proteins. Nucleic acid hybridization and polymerase chain reaction as sensitive detection methods. DNA sequencing. Analysis of genomes and proteomes by bioinformatics tools. Genome-wide analysis using microarrays.29. Applied Biotechnology and Bioremediations ES - 229Practical aspects of genetic engineering with microorganisms from extreme environment: Use of extremophilic microorganisms in waste treatment and methane production from agro industrial wastes; Production of enzymes like cellulase, proteases, amylases; alcohol and acetic acid production; Biocomposting: Microbial process involvement, vermin composting, biofertilizer, biopesticides production. Biomining: Microbial leaching of low grade mineral ores, molecular probes for organisms in mines and mine tailings, Petroleum pollutant biodegradation. Alternate fuels: Source and mechanism of various biofuel production. Bioremediation: Concept, role of bioremediation in controlling various pollution problems e.g. solid water, sewage water, industrial effluents, heavy metals, radioactive substances, oil spillage. Phytoremediation: Abatement of different types of pollution using plants, types of phytoremediation, mechanism involved with case studies. Waste water treatment strategies: Domestic and Industrial waste-water, application of microbiology waste treatment. Metagenomics: Environmental Genomics, ecogenomics or community genomics, the study of genetic material recovered directly from environmental samples and future applications in bioremediation.30. Eco-Toxicology ES - 230Principles in toxicology; Definition of Xenobiotics. Animal management in toxicological evaluation; Animal toxicity tests; Statistical concepts of LD50; Dose-effect and dose response relationship; Frequency response and cumulative response; Biological and chemical factors that influence toxicity; Bio-transformation and bio-accumulation. Influence of ecological factors on the effects of toxicity; Concept of green chemistry. Pollution of the ecosphere by industries; Global dispersion of toxic substance; Dispersion and circulating mechanisms of pollutants; degradable and non-degradable toxic substances; food chain. Eco-system influence on the fate and transport of toxicants. Aquatic toxicity tests; Statistical tests; Response of planktons to toxicants; EC49; Photosynthetic bacteria; Bio-absorption of heavy metals. Information management system in eco-toxicology.31. Environmental and Occupational Health ES - 231Basic principle of environmental health. Physiological responses of man to relevant stresses in the environment. Cases and effects of pollution. Industrial Toxicology: Study of environmental dose effect relationships. Evaluation of toxicity and threshold limits. Principles and methods of occupational health. The relationship of occupation of hygiene and safety and disease. Health maintenance: Survey, analysis and recommendations regarding health and safety problems in the working and living environment. Biostatistics, epidemiology: Application of statistical methods to medical records in the study of health problems of human population in a given environment. Treatment of variation, with demographic, vital statistics and epidemiological data. Hazard evaluation in polluted environment with specific emphasis on radiological health. Industrial hygiene technology-laboratory remains illustrating the principles, methods of recognizing evaluating and controlling environmental hazards like air pollution, etc.I would suggest you to visit the link School of Environmental Sciences for further information.
What is the meaning of life in three sentences?
I already gave the spiritual definition of life. It is too hard to express the life as a single sentence or a bunch of sentence it has its uniqueness on its own way.. so today I write what is life in biological way..Life is a characteristic that distinguishes physical entities that have biological processes, such as signaling and self-sustaining processes, from those that do not, either because such functions have ceased (they have died), or because they never had such functions and are classified as inanimate. Various forms of life exist, such as plants, animals, fungi, protists, archaea, and bacteria. The criteria can at times be ambiguous and may or may not define viruses, viroids, or potential synthetic life as "living". Biology is the science concerned with the study of life.LifePlants in the Rwenzori Mountains, UgandaScientific classificationDomains and SupergroupsLife on Earth:Non-cellular life[note 1] [note 2]Viruses[note 3]ViroidsCellular lifeDomain BacteriaDomain ArchaeaDomain EukaryaArchaeplastidaSARExcavataAmoebozoaOpisthokontaThere is currently no consensus regarding the definition of life. One popular definition is that organisms are open systems that maintain homeostasis, are composed of cells, have a life cycle, undergo metabolism, can grow, adapt to their environment, respond to stimuli, reproduce and evolve. However, several other definitions have been proposed, and there are some borderline cases of life, such as viruses or viroids.Abiogenesis attempts to describe the natural process of life arising from non-living matter, such as simple organic compounds. The prevailing scientific hypothesis is that the transition from non-living to living entities was not a single event, but a gradual process of increasing complexity. Life on Earth first appeared as early as 4.28 billion years ago, soon after ocean formation 4.41 billion years ago, and not long after the formation of the Earth 4.54 billion years ago.[1][2][3][4]The earliest known life forms are microfossils of bacteria.[5][6]Earth's current life may have descended from an RNA world, although RNA-based life may not have been the first. The mechanism by which life began on Earth is unknown, though many hypotheses have been formulated and are often based on the Miller–Urey experiment.Since its primordial beginnings, life on Earth has changed its environment on a geologic time scale, but it has also adapted to survive in most ecosystems and conditions. Some microorganisms, called extremophiles, thrive in physically or geochemically extreme environments that are detrimental to most other life on Earth. The cell is considered the structural and functional unit of life.[7]There are two kinds of cells, prokaryotic and eukaryotic, both of which consist of cytoplasm enclosed within a membrane and contain many biomolecules such as proteinsand nucleic acids. Cells reproduce through a process of cell division, in which the parent cell divides into two or more daughter cells.In the past, there have been many attempts to define what is meant by "life" through obsolete concepts such as odic force, hylomorphism, spontaneous generation and vitalism, that have now been disproved by biological discoveries. Aristotle was the first person to classify organisms. Later, Carl Linnaeus introduced his system of binomial nomenclature for the classification of species. Eventually new groups and categories of life were discovered, such as cells and microorganisms, forcing dramatic revisions of the structure of relationships between living organisms. Though currently only known on Earth, life need not be restricted to it, and many scientists speculate in the existence of extraterrestrial life. Artificial life is a computer simulation or man-made reconstruction of any aspect of life, which is often used to examine systems related to natural life.Death is the permanent termination of all biological functions which sustain an organism, and as such, is the end of its life. Extinction is the term describing the dying out of a group or taxon, usually a species. Fossilsare the preserved remains or traces of organisms.DefinitionsThe definition of life has long been a challenge for scientists and philosophers, with many varied definitions put forward.[8][9][10]This is partially because life is a process, not a substance.[11][12][13]This is complicated by a lack of knowledge of the characteristics of living entities, if any, that may have developed outside of Earth.[14][15]Philosophical definitions of life have also been put forward, with similar difficulties on how to distinguish living things from the non-living.[16]Legal definitions of life have also been described and debated, though these generally focus on the decision to declare a human dead, and the legal ramifications of this decision.[17]BiologySee also: OrganismThe characteristics of lifeSince there is no unequivocal definition of life, most current definitions in biology are descriptive. Life is considered a characteristic of something that preserves, furthers or reinforces its existence in the given environment. This characteristic exhibits all or most of the following traits:[10][18][19][20][21][22][23]Homeostasis: regulation of the internal environment to maintain a constant state; for example, sweating to reduce temperatureOrganization: being structurally composed of one or more cells – the basic units of lifeMetabolism: transformation of energy by converting chemicals and energy into cellular components (anabolism) and decomposing organic matter (catabolism). Living things require energy to maintain internal organization (homeostasis) and to produce the other phenomena associated with life.Growth: maintenance of a higher rate of anabolism than catabolism. A growing organism increases in size in all of its parts, rather than simply accumulating matter.Adaptation: the ability to change over time in response to the environment. This ability is fundamental to the process of evolution and is determined by the organism's heredity, diet, and external factors.Response to stimuli: a response can take many forms, from the contraction of a unicellular organism to external chemicals, to complex reactions involving all the senses of multicellular organisms. A response is often expressed by motion; for example, the leaves of a plant turning toward the sun (phototropism), and chemotaxis.Reproduction: the ability to produce new individual organisms, either asexually from a single parent organism or sexually from two parent organisms.These complex processes, called physiological functions, have underlying physical and chemical bases, as well as signaling and control mechanisms that are essential to maintaining life.Alternative definitionsSee also: Entropy and lifeFrom a physics perspective, living beings are thermodynamic systems with an organized molecular structure that can reproduce itself and evolve as survival dictates.[24][25]Thermodynamically, life has been described as an open system which makes use of gradients in its surroundings to create imperfect copies of itself.[26]Hence, life is a self-sustained chemical system capable of undergoing Darwinian evolution.[27][28]A major strength of this definition is that it distinguishes life by the evolutionary process rather than its chemical composition.[29]Others take a systemic viewpoint that does not necessarily depend on molecular chemistry. One systemic definition of life is that living things are self-organizing and autopoietic (self-producing). Variations of this definition include Stuart Kauffman's definition as an autonomous agent or a multi-agent system capable of reproducing itself or themselves, and of completing at least one thermodynamic work cycle.[30]This definition is extended by the apparition of novel functions over time.[31]VirusesMain article: VirusAdenovirus as seen under an electron microscopeWhether or not viruses should be considered as alive is controversial. They are most often considered as just replicators rather than forms of life.[32]They have been described as "organisms at the edge of life"[33]because they possess genes, evolve by natural selection,[34][35]and replicate by creating multiple copies of themselves through self-assembly. However, viruses do not metabolize and they require a host cell to make new products. Virus self-assembly within host cells has implications for the study of the origin of life, as it may support the hypothesis that life could have started as self-assembling organic molecules.[36][37][38]BiophysicsTo reflect the minimum phenomena required, other biological definitions of life have been proposed,[39]with many of these being based upon chemical systems. Biophysicists have commented that living things function on negative entropy.[40][41]In other words, living processes can be viewed as a delay of the spontaneous diffusion or dispersion of the internal energy of biological moleculestowards more potential microstates.[8]In more detail, according to physicists such as John Bernal, Erwin Schrödinger, Eugene Wigner, and John Avery, life is a member of the class of phenomena that are open or continuous systems able to decrease their internal entropy at the expense of substances or free energy taken in from the environment and subsequently rejected in a degraded form.[42][43]Living systems theoriesLiving systems are open self-organizing living things that interact with their environment. These systems are maintained by flows of information, energy, and matter.Some scientists have proposed in the last few decades that a general living systems theory is required to explain the nature of life.[44]Such a general theory would arise out of the ecological and biological sciences and attempt to map general principles for how all living systems work. Instead of examining phenomena by attempting to break things down into components, a general living systems theory explores phenomena in terms of dynamic patterns of the relationships of organisms with their environment.[45]Gaia hypothesisMain article: Gaia hypothesisThe idea that the Earth is alive is found in philosophy and religion, but the first scientific discussion of it was by the Scottish scientist James Hutton. In 1785, he stated that the Earth was a superorganism and that its proper study should be physiology. Hutton is considered the father of geology, but his idea of a living Earth was forgotten in the intense reductionism of the 19th century.[46]:10The Gaia hypothesis, proposed in the 1960s by scientist James Lovelock,[47][48]suggests that life on Earth functions as a single organism that defines and maintains environmentalconditions necessary for its survival.[46]This hypothesis served as one of the foundations of the modern Earth system science.NonfractionabilityThe first attempt at a general living systemstheory for explaining the nature of life was in 1978, by American biologist James Grier Miller.[49]Robert Rosen (1991) built on this by defining a system component as "a unit of organization; a part with a function, i.e., a definite relation between part and whole." From this and other starting concepts, he developed a "relational theory of systems" that attempts to explain the special properties of life. Specifically, he identified the "nonfractionability of components in an organism" as the fundamental difference between living systems and "biological machines."[50]Life as a property of ecosystemsA systems view of life treats environmental fluxes and biological fluxes together as a "reciprocity of influence,"[51]and a reciprocal relation with environment is arguably as important for understanding life as it is for understanding ecosystems. As Harold J. Morowitz (1992) explains it, life is a property of an ecological system rather than a single organism or species.[52]He argues that an ecosystemic definition of life is preferable to a strictly biochemical or physical one. Robert Ulanowicz (2009) highlights mutualism as the key to understand the systemic, order-generating behavior of life and ecosystems.[53]Complex systems biologyMain article: Complex systems biologySee also: Mathematical biologyComplex systems biology (CSB) is a field of science that studies the emergence of complexity in functional organisms from the viewpoint of dynamic systems theory.[54]The latter is also often called systems biology and aims to understand the most fundamental aspects of life. A closely related approach to CSB and systems biology called relational biology is concerned mainly with understanding life processes in terms of the most important relations, and categories of such relations among the essential functional components of organisms; for multicellular organisms, this has been defined as "categorical biology", or a model representation of organisms as a category theory of biological relations, as well as an algebraic topology of the functional organization of living organisms in terms of their dynamic, complex networks of metabolic, genetic, and epigenetic processes and signaling pathways.[55][56]Alternative but closely related approaches focus on the interdependance of constraints, where constraints can be either molecular, such as enzymes, or macroscopic, such as the geometry of a bone or of the vascular system.[57]Darwinian dynamicIt has also been argued that the evolution of order in living systems and certain physical systems obeys a common fundamental principle termed the Darwinian dynamic.[58][59]The Darwinian dynamic was formulated by first considering how macroscopic order is generated in a simple non-biological system far from thermodynamic equilibrium, and then extending consideration to short, replicating RNA molecules. The underlying order-generating process was concluded to be basically similar for both types of systems.[58]Operator theoryAnother systemic definition called the operator theory proposes that "life is a general term for the presence of the typical closures found in organisms; the typical closures are a membrane and an autocatalytic set in the cell"[60]and that an organism is any system with an organisation that complies with an operator type that is at least as complex as the cell.[61][62][63][64]Life can also be modeled as a network of inferior negative feedbacks of regulatory mechanisms subordinated to a superior positive feedback formed by the potential of expansion and reproduction.[65]History of studyMaterialismMain article: MaterialismPlant growth in the Hoh RainforestHerds of zebra and impala gathering on the Maasai MaraplainAn aerial photo of microbial mats around the Grand Prismatic Spring of Yellowstone National ParkSome of the earliest theories of life were materialist, holding that all that exists is matter, and that life is merely a complex form or arrangement of matter. Empedocles (430 BC) argued that everything in the universe is made up of a combination of four eternal "elements" or "roots of all": earth, water, air, and fire. All change is explained by the arrangement and rearrangement of these four elements. The various forms of life are caused by an appropriate mixture of elements.[66]Democritus (460 BC) thought that the essential characteristic of life is having a soul(psyche). Like other ancient writers, he was attempting to explain what makes something a living thing. His explanation was that fiery atoms make a soul in exactly the same way atoms and void account for any other thing. He elaborates on fire because of the apparent connection between life and heat, and because fire moves.[67]Plato's world of eternal and unchanging Forms, imperfectly represented in matter by a divine Artisan, contrasts sharply with the various mechanistic Weltanschauungen, of which atomism was, by the fourth century at least, the most prominent ... This debate persisted throughout the ancient world. Atomistic mechanism got a shot in the arm from Epicurus ... while the Stoics adopted a divine teleology ... The choice seems simple: either show how a structured, regular world could arise out of undirected processes, or inject intelligence into the system.[68]—R.J. Hankinson, Cause and Explanation in Ancient Greek ThoughtThe mechanistic materialism that originated in ancient Greece was revived and revised by the French philosopher René Descartes, who held that animals and humans were assemblages of parts that together functioned as a machine. In the 19th century, the advances in cell theory in biological science encouraged this view. The evolutionary theory of Charles Darwin (1859) is a mechanistic explanation for the origin of species by means of natural selection.[69]HylomorphismMain article: HylomorphismThe structure of the souls of plants, animals, and humans, according to AristotleHylomorphism is a theory first expressed by the Greek philosopher Aristotle (322 BC). The application of hylomorphism to biology was important to Aristotle, and biology is extensively covered in his extant writings. In this view, everything in the material universe has both matter and form, and the form of a living thing is its soul (Greek psyche, Latin anima). There are three kinds of souls: the vegetative soul of plants, which causes them to grow and decay and nourish themselves, but does not cause motion and sensation; the animal soul, which causes animals to move and feel; and the rational soul, which is the source of consciousness and reasoning, which (Aristotle believed) is found only in man.[70]Each higher soul has all of the attributes of the lower ones. Aristotle believed that while matter can exist without form, form cannot exist without matter, and that therefore the soul cannot exist without the body.[71]This account is consistent with teleologicalexplanations of life, which account for phenomena in terms of purpose or goal-directedness. Thus, the whiteness of the polar bear's coat is explained by its purpose of camouflage. The direction of causality (from the future to the past) is in contradiction with the scientific evidence for natural selection, which explains the consequence in terms of a prior cause. Biological features are explained not by looking at future optimal results, but by looking at the past evolutionary history of a species, which led to the natural selection of the features in question.[72]Spontaneous generationMain article: Spontaneous generationSpontaneous generation was the belief that living organisms can form without descent from similar organisms. Typically, the idea was that certain forms such as fleas could arise from inanimate matter such as dust or the supposed seasonal generation of mice and insects from mud or garbage.[73]The theory of spontaneous generation was proposed by Aristotle,[74]who compiled and expanded the work of prior natural philosophers and the various ancient explanations of the appearance of organisms; it held sway for two millennia. It was decisively dispelled by the experiments of Louis Pasteur in 1859, who expanded upon the investigations of predecessors such as Francesco Redi.[75][76]Disproof of the traditional ideas of spontaneous generation is no longer controversial among biologists.[77][78][79]VitalismMain article: VitalismVitalism is the belief that the life-principle is non-material. This originated with Georg Ernst Stahl (17th century), and remained popular until the middle of the 19th century. It appealed to philosophers such as Henri Bergson, Friedrich Nietzsche, and Wilhelm Dilthey,[80]anatomists like Marie François Xavier Bichat, and chemists like Justus von Liebig.[81]Vitalism included the idea that there was a fundamental difference between organic and inorganic material, and the belief that organic material can only be derived from living things. This was disproved in 1828, when Friedrich Wöhler prepared urea from inorganic materials.[82]This Wöhler synthesisis considered the starting point of modern organic chemistry. It is of historical significance because for the first time an organic compound was produced in inorganicreactions.[81]During the 1850s, Hermann von Helmholtz, anticipated by Julius Robert von Mayer, demonstrated that no energy is lost in muscle movement, suggesting that there were no "vital forces" necessary to move a muscle.[83]These results led to the abandonment of scientific interest in vitalistic theories, although the belief lingered on in pseudoscientific theories such as homeopathy, which interprets diseases and sickness as caused by disturbances in a hypothetical vital force or life force.[84]OriginThe age of the Earth is about 4.54 billion years.[85][86][87] Evidence suggests that life on Earth has existed for at least 3.5 billion years,[88][89][90][91][92][93][94][95][96] with the oldest physical traces of life dating back 3.7 billion years;[97][98][99] however, some theories, such as the Late Heavy Bombardment theory, suggest that life on Earth may have started even earlier, as early as 4.1–4.4 billion years ago,[88][89][90][91][92] and the chemistry leading to life may have begun shortly after the Big Bang, 13.8 billion years ago, during an epoch when the universe was only 10–17 million years old.[100][101][102]More than 99% of all species of life forms, amounting to over five billion species,[103] that ever lived on Earth are estimated to be extinct.[104][105]Although the number of Earth's catalogued species of lifeforms is between 1.2 million and 2 million,[106][107] the total number of species in the planet is uncertain. Estimates range from 8 million to 100 million,[106][107] with a more narrow range between 10 and 14 million,[106] but it may be as high as 1 trillion (with only one-thousandth of one percent of the species described) according to studies realized in May 2016.[108][109] The total number of related DNA base pairs on Earth is estimated at 5.0 x 1037 and weighs 50 billion tonnes.[110] In comparison, the total mass of the biosphere has been estimated to be as much as 4 TtC (trillion tons of carbon).[111] In July 2016, scientists reported identifying a set of 355 genes from the Last Universal Common Ancestor (LUCA) of all organisms living on Earth.[112]All known life forms share fundamental molecular mechanisms, reflecting their common descent; based on these observations, hypotheses on the origin of life attempt to find a mechanism explaining the formation of a universal common ancestor, from simple organic molecules via pre-cellular life to protocells and metabolism. Models have been divided into "genes-first" and "metabolism-first" categories, but a recent trend is the emergence of hybrid models that combine both categories.[113]There is no current scientific consensus as to how life originated. However, most accepted scientific models build on the Miller–Urey experiment and the work of Sidney Fox, which show that conditions on the primitive Earth favored chemical reactions that synthesize amino acids and other organic compounds from inorganic precursors,[114] and phospholipids spontaneously form lipid bilayers, the basic structure of a cell membrane.Living organisms synthesize proteins, which are polymers of amino acids using instructions encoded by deoxyribonucleic acid (DNA). Protein synthesis entails intermediary ribonucleic acid (RNA) polymers. One possibility for how life began is that genes originated first, followed by proteins;[115] the alternative being that proteins came first and then genes.[116]However, because genes and proteins are both required to produce the other, the problem of considering which came first is like that of the chicken or the egg. Most scientists have adopted the hypothesis that because of this, it is unlikely that genes and proteins arose independently.[117]Therefore, a possibility, first suggested by Francis Crick,[118] is that the first life was based on RNA,[117] which has the DNA-like properties of information storage and the catalytic properties of some proteins. This is called the RNA world hypothesis, and it is supported by the observation that many of the most critical components of cells (those that evolve the slowest) are composed mostly or entirely of RNA. Also, many critical cofactors (ATP, Acetyl-CoA, NADH, etc.) are either nucleotides or substances clearly related to them. The catalytic properties of RNA had not yet been demonstrated when the hypothesis was first proposed,[119] but they were confirmed by Thomas Cech in 1986.[120]One issue with the RNA world hypothesis is that synthesis of RNA from simple inorganic precursors is more difficult than for other organic molecules. One reason for this is that RNA precursors are very stable and react with each other very slowly under ambient conditions, and it has also been proposed that living organisms consisted of other molecules before RNA.[121] However, the successful synthesis of certain RNA molecules under the conditions that existed prior to life on Earth has been achieved by adding alternative precursors in a specified order with the precursor phosphate present throughout the reaction.[122] This study makes the RNA world hypothesis more plausible.[123]Geological findings in 2013 showed that reactive phosphorus species (like phosphite) were in abundance in the ocean before 3.5 Ga, and that Schreibersite easily reacts with aqueous glycerol to generate phosphite and glycerol 3-phosphate.[124] It is hypothesized that Schreibersite-containing meteorites from the Late Heavy Bombardment could have provided early reduced phosphorus, which could react with prebiotic organic molecules to form phosphorylated biomolecules, like RNA.[124]In 2009, experiments demonstrated Darwinian evolution of a two-component system of RNA enzymes (ribozymes) in vitro.[125] The work was performed in the laboratory of Gerald Joyce, who stated "This is the first example, outside of biology, of evolutionary adaptation in a molecular genetic system."[126]Prebiotic compounds may have originated extraterrestrially. NASA findings in 2011, based on studies with meteorites found on Earth, suggest DNA and RNA components (adenine, guanine and related organic molecules) may be formed in outer space.[127][128][129][130]In March 2015, NASA scientists reported that, for the first time, complex DNA and RNA organic compounds of life, including uracil, cytosine and thymine, have been formed in the laboratory under outer space conditions, using starting chemicals, such as pyrimidine, found in meteorites. Pyrimidine, like polycyclic aromatic hydrocarbons (PAHs), the most carbon-rich chemical found in the universe, may have been formed in red giants or in interstellar dust and gas clouds, according to the scientists.[131]According to the panspermia hypothesis, microscopic life—distributed by meteoroids, asteroids and other small Solar System bodies—may exist throughout the universe.[132]Environmental conditionsCyanobacteria dramatically changed the composition of life forms on Earth by leading to the near-extinction of oxygen-intolerant organisms.The diversity of life on Earth is a result of the dynamic interplay between genetic opportunity, metabolic capability, environmental challenges,[133] and symbiosis.[134][135][136] For most of its existence, Earth's habitable environment has been dominated by microorganisms and subjected to their metabolism and evolution. As a consequence of these microbial activities, the physical-chemical environment on Earth has been changing on a geologic time scale, thereby affecting the path of evolution of subsequent life.[133] For example, the release of molecular oxygen by cyanobacteria as a by-product of photosynthesis induced global changes in the Earth's environment. Because oxygen was toxic to most life on Earth at the time, this posed novel evolutionary challenges, and ultimately resulted in the formation of Earth's major animal and plant species. This interplay between organisms and their environment is an inherent feature of living systems.[133]BiosphereMain article: BiosphereThe biosphere is the global sum of all ecosystems. It can also be termed as the zone of life on Earth, a closed system (apart from solar and cosmic radiation and heat from the interior of the Earth), and largely self-regulating.[137] By the most general biophysiological definition, the biosphere is the global ecological system integrating all living beings and their relationships, including their interaction with the elements of the lithosphere, geosphere, hydrosphere, and atmosphere.Life forms live in every part of the Earth's biosphere, including soil, hot springs, inside rocks at least 19 km (12 mi) deep underground, the deepest parts of the ocean, and at least 64 km (40 mi) high in the atmosphere.[138][139][140] Under certain test conditions, life forms have been observed to thrive in the near-weightlessness of space[141][142] and to survive in the vacuum of outer space.[143][144] Life forms appear to thrive in the Mariana Trench, the deepest spot in the Earth's oceans.[145][146] Other researchers reported related studies that life forms thrive inside rocks up to 580 m (1,900 ft; 0.36 mi) below the sea floor under 2,590 m (8,500 ft; 1.61 mi) of ocean off the coast of the northwestern United States,[145][147] as well as 2,400 m (7,900 ft; 1.5 mi) beneath the seabed off Japan.[148] In August 2014, scientists confirmed the existence of life forms living 800 m (2,600 ft; 0.50 mi) below the ice of Antarctica.[149][150] According to one researcher, "You can find microbes everywhere—they're extremely adaptable to conditions, and survive wherever they are."[145]The biosphere is postulated to have evolved, beginning with a process of biopoesis (life created naturally from non-living matter, such as simple organic compounds) or biogenesis (life created from living matter), at least some 3.5 billion years ago.[151][152] The earliest evidence for life on Earth includes biogenic graphite found in 3.7 billion-year-old metasedimentary rocks from Western Greenland[97] and microbial mat fossils found in 3.48 billion-year-old sandstone from Western Australia.[98][99] More recently, in 2015, "remains of biotic life" were found in 4.1 billion-year-old rocks in Western Australia.[89][90] In 2017, putative fossilized microorganisms (or microfossils) were announced to have been discovered in hydrothermal vent precipitates in the Nuvvuagittuq Belt of Quebec, Canada that were as old as 4.28 billion years, the oldest record of life on earth, suggesting "an almost instantaneous emergence of life" after ocean formation 4.4 billion years ago, and not long after the formation of the Earth 4.54 billion years ago.[1][2][3][4] According to biologist Stephen Blair Hedges, "If life arose relatively quickly on Earth ... then it could be common in the universe."[89]In a general sense, biospheres are any closed, self-regulating systems containing ecosystems. This includes artificial biospheres such as Biosphere 2 and BIOS-3, and potentially ones on other planets or moons.[153]Range of toleranceDeinococcus radiodurans is an extremophile that can resist extremes of cold, dehydration, vacuum, acid, and radiation exposure.The inert components of an ecosystem are the physical and chemical factors necessary for life—energy (sunlight or chemical energy), water, heat, atmosphere, gravity, nutrients, and ultraviolet solar radiation protection.[154] In most ecosystems, the conditions vary during the day and from one season to the next. To live in most ecosystems, then, organisms must be able to survive a range of conditions, called the "range of tolerance."[155] Outside that are the "zones of physiological stress," where the survival and reproduction are possible but not optimal. Beyond these zones are the "zones of intolerance," where survival and reproduction of that organism is unlikely or impossible. Organisms that have a wide range of tolerance are more widely distributed than organisms with a narrow range of tolerance.[155]ExtremophilesFurther information: ExtremophileTo survive, selected microorganisms can assume forms that enable them to withstand freezing, complete desiccation, starvation, high levels of radiation exposure, and other physical or chemical challenges. These microorganisms may survive exposure to such conditions for weeks, months, years, or even centuries.[133] Extremophiles are microbial life forms that thrive outside the ranges where life is commonly found.[156] They excel at exploiting uncommon sources of energy. While all organisms are composed of nearly identical molecules, evolution has enabled such microbes to cope with this wide range of physical and chemical conditions. Characterization of the structure and metabolic diversity of microbial communities in such extreme environments is ongoing.[157]Microbial life forms thrive even in the Mariana Trench, the deepest spot in the Earth's oceans.[145][146] Microbes also thrive inside rocks up to 1,900 feet (580 m) below the sea floor under 8,500 feet (2,600 m) of ocean.[145][147]Investigation of the tenacity and versatility of life on Earth,[156] as well as an understanding of the molecular systems that some organisms utilize to survive such extremes, is important for the search for life beyond Earth.[133] For example, lichen could survive for a month in a simulated Martian environment.[158][159]Chemical elementsAll life forms require certain core chemical elements needed for biochemical functioning. These include carbon, hydrogen, nitrogen, oxygen, phosphorus, and sulfur—the elemental macronutrients for all organisms[160]—often represented by the acronym CHNOPS. Together these make up nucleic acids, proteins and lipids, the bulk of living matter. Five of these six elements comprise the chemical components of DNA, the exception being sulfur. The latter is a component of the amino acids cysteine and methionine. The most biologically abundant of these elements is carbon, which has the desirable attribute of forming multiple, stable covalent bonds. This allows carbon-based (organic) molecules to form an immense variety of chemical arrangements.[161] Alternative hypothetical types of biochemistry have been proposed that eliminate one or more of these elements, swap out an element for one not on the list, or change required chiralities or other chemical properties.[162][163]DNAMain article: DNADeoxyribonucleic acid is a molecule that carries most of the genetic instructions used in the growth, development, functioning and reproduction of all known living organisms and many viruses. DNA and RNA are nucleic acids; alongside proteins and complex carbohydrates, they are one of the three major types of macromolecule that are essential for all known forms of life. Most DNA molecules consist of two biopolymer strands coiled around each other to form a double helix. The two DNA strands are known as polynucleotides since they are composed of simpler units called nucleotides.[164] Each nucleotide is composed of a nitrogen-containing nucleobase—either cytosine (C), guanine (G), adenine (A), or thymine (T)—as well as a sugar called deoxyribose and a phosphate group. The nucleotides are joined to one another in a chain by covalent bonds between the sugar of one nucleotide and the phosphate of the next, resulting in an alternating sugar-phosphate backbone. According to base pairing rules (A with T, and C with G), hydrogen bonds bind the nitrogenous bases of the two separate polynucleotide strands to make double-stranded DNA. The total amount of related DNA base pairs on Earth is estimated at 5.0 x 1037, and weighs 50 billion tonnes.[110] In comparison, the total mass of the biosphere has been estimated to be as much as 4 TtC (trillion tons of carbon).[111]DNA stores biological information. The DNA backbone is resistant to cleavage, and both strands of the double-stranded structure store the same biological information. Biological information is replicated as the two strands are separated. A significant portion of DNA (more than 98% for humans) is non-coding, meaning that these sections do not serve as patterns for protein sequences.The two strands of DNA run in opposite directions to each other and are therefore anti-parallel. Attached to each sugar is one of four types of nucleobases (informally, bases). It is the sequence of these four nucleobases along the backbone that encodes biological information. Under the genetic code, RNA strands are translated to specify the sequence of amino acids within proteins. These RNA strands are initially created using DNA strands as a template in a process called transcription.Within cells, DNA is organized into long structures called chromosomes. During cell division these chromosomes are duplicated in the process of DNA replication, providing each cell its own complete set of chromosomes. Eukaryotic organisms (animals, plants, fungi, and protists) store most of their DNA inside the cell nucleus and some of their DNA in organelles, such as mitochondria or chloroplasts.[165] In contrast, prokaryotes (bacteria and archaea) store their DNA only in the cytoplasm. Within the chromosomes, chromatin proteins such as histones compact and organize DNA. These compact structures guide the interactions between DNA and other proteins, helping control which parts of the DNA are transcribed.DNA was first isolated by Friedrich Miescher in 1869.[166] Its molecular structure was identified by James Watson and Francis Crick in 1953, whose model-building efforts were guided by X-ray diffraction data acquired by Rosalind Franklin.[167]ClassificationMain article: Biological classificationThe hierarchy of biological classification's eight major taxonomic ranks. Life is divided into domains, which are subdivided into further groups. Intermediate minor rankings are not shown.AntiquityThe first known attempt to classify organisms was conducted by the Greek philosopher Aristotle (384–322 BC), who classified all living organisms known at that time as either a plant or an animal, based mainly on their ability to move. He also distinguished animals with blood from animals without blood (or at least without red blood), which can be compared with the concepts of vertebrates and invertebrates respectively, and divided the blooded animals into five groups: viviparous quadrupeds (mammals), oviparous quadrupeds (reptiles and amphibians), birds, fishes and whales. The bloodless animals were also divided into five groups: cephalopods, crustaceans, insects (which included the spiders, scorpions, and centipedes, in addition to what we define as insects today), shelled animals (such as most molluscs and echinoderms), and "zoophytes" (animals that resemble plants). Though Aristotle's work in zoology was not without errors, it was the grandest biological synthesis of the time and remained the ultimate authority for many centuries after his death.[168]LinnaeanThe exploration of the Americas revealed large numbers of new plants and animals that needed descriptions and classification. In the latter part of the 16th century and the beginning of the 17th, careful study of animals commenced and was gradually extended until it formed a sufficient body of knowledge to serve as an anatomical basis for classification.In the late 1740s, Carl Linnaeus introduced his system of binomial nomenclature for the classification of species. Linnaeus attempted to improve the composition and reduce the length of the previously used many-worded names by abolishing unnecessary rhetoric, introducing new descriptive terms and precisely defining their meaning.[169] The Linnaean classification has eight levels: domains, kingdoms, phyla, class, order, family, genus, and species.The fungi were originally treated as plants. For a short period Linnaeus had classified them in the taxon Vermes in Animalia, but later placed them back in Plantae. Copeland classified the Fungi in his Protoctista, thus partially avoiding the problem but acknowledging their special status.[170] The problem was eventually solved by Whittaker, when he gave them their own kingdom in his five-kingdom system. Evolutionary history shows that the fungi are more closely related to animals than to plants.[171]As new discoveries enabled detailed study of cells and microorganisms, new groups of life were revealed, and the fields of cell biology and microbiology were created. These new organisms were originally described separately in protozoa as animals and protophyta/thallophyta as plants, but were united by Haeckel in the kingdom Protista; later, the prokaryotes were split off in the kingdom Monera, which would eventually be divided into two separate groups, the Bacteria and the Archaea. This led to the six-kingdom system and eventually to the current three-domain system, which is based on evolutionary relationships.[172] However, the classification of eukaryotes, especially of protists, is still controversial.[173]As microbiology, molecular biology and virology developed, non-cellular reproducing agents were discovered, such as viruses and viroids. Whether these are considered alive has been a matter of debate; viruses lack characteristics of life such as cell membranes, metabolism and the ability to grow or respond to their environments. Viruses can still be classed into "species" based on their biology and genetics, but many aspects of such a classification remain controversial.[174]In May 2016, scientists reported that 1 trillion species are estimated to be on Earth currently with only one-thousandth of one percent described.[108]The original Linnaean system has been modified over time as follows:Linnaeus1735[175] Haeckel1866[176] Chatton1925[177] Copeland1938[178] Whittaker1969[179] Woese et al.1990[172] Cavalier-Smith1998[180] Cavalier-Smith2015[181]2 kingdoms 3 kingdoms 2 empires 4 kingdoms 5 kingdoms 3 domains 2 empires, 6 kingdoms 2 empires, 7 kingdoms(not treated) Protista Prokaryota Monera Monera Bacteria Bacteria BacteriaArchaea ArchaeaEukaryota Protoctista Protista Eucarya Protozoa ProtozoaChromista ChromistaVegetabilia Plantae Plantae Plantae Plantae PlantaeFungi Fungi FungiAnimalia Animalia Animalia Animalia Animalia AnimaliaMain article: Kingdom (biology) § SummaryCladisticIn the 1960s cladistics emerged: a system arranging taxa based on clades in an evolutionary or phylogenetic tree.[182]CellsMain article: Cell (biology)Cells are the basic unit of structure in every living thing, and all cells arise from pre-existing cells by division. Cell theory was formulated by Henri Dutrochet, Theodor Schwann, Rudolf Virchow and others during the early nineteenth century, and subsequently became widely accepted.[183] The activity of an organism depends on the total activity of its cells, with energy flow occurring within and between them.[184] Cells contain hereditary information that is carried forward as a genetic code during cell division.[185]There are two primary types of cells. Prokaryotes lack a nucleus and other membrane-bound organelles, although they have circular DNA and ribosomes. Bacteria and Archaea are two domains of prokaryotes. The other primary type of cells are the eukaryotes, which have distinct nuclei bound by a nuclear membrane and membrane-bound organelles, including mitochondria, chloroplasts, lysosomes, rough and smooth endoplasmic reticulum, and vacuoles. In addition, they possess organized chromosomes that store genetic material. All species of large complex organisms are eukaryotes, including animals, plants and fungi, though most species of eukaryote are protist microorganisms.[186] The conventional model is that eukaryotes evolved from prokaryotes, with the main organelles of the eukaryotes forming through endosymbiosis between bacteria and the progenitor eukaryotic cell.[187]The molecular mechanisms of cell biology are based on proteins. Most of these are synthesized by the ribosomes through an enzyme-catalyzed process called protein biosynthesis. A sequence of amino acids is assembled and joined together based upon gene expression of the cell's nucleic acid.[188] In eukaryotic cells, these proteins may then be transported and processed through the Golgi apparatus in preparation for dispatch to their destination.[189]Cells reproduce through a process of cell division in which the parent cell divides into two or more daughter cells. For prokaryotes, cell division occurs through a process of fission in which the DNA is replicated, then the two copies are attached to parts of the cell membrane. In eukaryotes, a more complex process of mitosis is followed. However, the end result is the same; the resulting cell copies are identical to each other and to the original cell (except for mutations), and both are capable of further division following an interphase period.[190]Multicellular organisms may have first evolved through the formation of colonies of identical cells. These cells can form group organisms through cell adhesion. The individual members of a colony are capable of surviving on their own, whereas the members of a true multi-cellular organism have developed specializations, making them dependent on the remainder of the organism for survival. Such organisms are formed clonally or from a single germ cell that is capable of forming the various specialized cells that form the adult organism. This specialization allows multicellular organisms to exploit resources more efficiently than single cells.[191] In January 2016, scientists reported that, about 800 million years ago, a minor genetic change in a single molecule, called GK-PID, may have allowed organisms to go from a single cell organism to one of many cells.[192]Cells have evolved methods to perceive and respond to their microenvironment, thereby enhancing their adaptability. Cell signaling coordinates cellular activities, and hence governs the basic functions of multicellular organisms. Signaling between cells can occur through direct cell contact using juxtacrine signalling, or indirectly through the exchange of agents as in the endocrine system. In more complex organisms, coordination of activities can occur through a dedicated nervous system.[193]ExtraterrestrialMain articles: Extraterrestrial life, Astrobiology, and AstroecologyThough life is confirmed only on Earth, many think that extraterrestrial life is not only plausible, but probable or inevitable.[194][195] Other planets and moons in the Solar System and other planetary systems are being examined for evidence of having once supported simple life, and projects such as SETI are trying to detect radio transmissions from possible alien civilizations. Other locations within the Solar System that may host microbial life include the subsurface of Mars, the upper atmosphere of Venus,[196] and subsurface oceans on some of the moons of the giant planets.[197][198] Beyond the Solar System, the region around another main-sequence star that could support Earth-like life on an Earth-like planet is known as the habitable zone. The inner and outer radii of this zone vary with the luminosity of the star, as does the time interval during which the zone survives. Stars more massive than the Sun have a larger habitable zone, but remain on the Sun-like "main sequence" of stellar evolution for a shorter time interval. Small red dwarfs have the opposite problem, with a smaller habitable zone that is subject to higher levels of magnetic activity and the effects of tidal locking from close orbits. Hence, stars in the intermediate mass range such as the Sun may have a greater likelihood for Earth-like life to develop.[199] The location of the star within a galaxy may also affect the likelihood of life forming. Stars in regions with a greater abundance of heavier elements that can form planets, in combination with a low rate of potentially habitat-damaging supernova events, are predicted to have a higher probability of hosting planets with complex life.[200] The variables of the Drake equation are used to discuss the conditions in planetary systems where civilization is most likely to exist.[201] Use of the equation to predict the amount of extraterrestrial life, however, is difficult; because many of the variables are unknown, the equation functions as more of a mirror to what its user already thinks. As a result, the number of civilizations in the galaxy can be estimated as low as 9.1 x 10−11 or as high as 156 million; for the calculations, see Drake equation.ArtificialMain articles: Artificial life and Synthetic biologyArtificial life is the simulation of any aspect of life, as through computers, robotics, or biochemistry.[202] The study of artificial life imitates traditional biology by recreating some aspects of biological phenomena. Scientists study the logic of living systems by creating artificial environments—seeking to understand the complex information processing that defines such systems.[184] While life is, by definition, alive, artificial life is generally referred to as data confined to a digital environment and existence.Synthetic biology is a new area of biotechnology that combines science and biological engineering. The common goal is the design and construction of new biological functions and systems not found in nature. Synthetic biology includes the broad redefinition and expansion of biotechnology, with the ultimate goals of being able to design and build engineered biological systems that process information, manipulate chemicals, fabricate materials and structures, produce energy, provide food, and maintain and enhance human health and the environment.[203]DeathMain article: DeathAnimal corpses, like this African buffalo, are recycled by the ecosystem, providing energy and nutrients for living creaturesDeath is the permanent termination of all vital functions or life processes in an organism or cell.[204][205] It can occur as a result of an accident, medical conditions, biological interaction, malnutrition, poisoning, senescence, or suicide. After death, the remains of an organism re-enter the biogeochemical cycle. Organisms may be consumed by a predator or a scavenger and leftover organic material may then be further decomposed by detritivores, organisms that recycle detritus, returning it to the environment for reuse in the food chain.One of the challenges in defining death is in distinguishing it from life. Death would seem to refer to either the moment life ends, or when the state that follows life begins.[205] However, determining when death has occurred is difficult, as cessation of life functions is often not simultaneous across organ systems.[206] Such determination therefore requires drawing conceptual lines between life and death. This is problematic, however, because there is little consensus over how to define life. The nature of death has for millennia been a central concern of the world's religious traditions and of philosophical inquiry. Many religions maintain faith in either a kind of afterlife or reincarnation for the soul, or resurrection of the body at a later date.ExtinctionMain article: ExtinctionExtinction is the process by which a group of taxa or species dies out, reducing biodiversity.[207] The moment of extinction is generally considered the death of the last individual of that species. Because a species' potential range may be very large, determining this moment is difficult, and is usually done retrospectively after a period of apparent absence. Species become extinct when they are no longer able to survive in changing habitat or against superior competition. In Earth's history, over 99% of all the species that have ever lived are extinct;[208][103][104][105] however, mass extinctions may have accelerated evolution by providing opportunities for new groups of organisms to diversify.[209]FossilsMain article: FossilsFossils are the preserved remains or traces of animals, plants, and other organisms from the remote past. The totality of fossils, both discovered and undiscovered, and their placement in fossil-containing rock formations and sedimentary layers (strata) is known as the fossil record. A preserved specimen is called a fossil if it is older than the arbitrary date of 10,000 years ago.[210] Hence, fossils range in age from the youngest at the start of the Holocene Epoch to the oldest from the Archaean Eon, up to 3.4 billion years old.[211][212]
Is it possible for anything to violate the laws of physics?
1 The placebo effectDon’t try this at home. Several times a day, for several days, you induce pain in someone. You control the pain with morphine until the final day of the experiment, when you replace the morphine with saline solution. Guess what? The saline takes the pain away.This is the placebo effect: somehow, sometimes, a whole lot of nothing can be very powerful. Except it’s not quite nothing. When Fabrizio Benedetti of the University of Turin in Italy carried out the above experiment, he added a final twist by adding naloxone, a drug that blocks the effects of morphine, to the saline. The shocking result? The pain-relieving power of saline solution disappeared.So what is going on? Doctors have known about the placebo effect for decades, and the naloxone result seems to show that the placebo effect is somehow biochemical. But apart from that, we simply don’t know.Benedetti has since shown that a saline placebo can also reduce tremors and muscle stiffness in people with Parkinson’s disease. He and his team measured the activity of neurons in the patients’ brains as they administered the saline. They found that individual neurons in the subthalamic nucleus (a common target for surgical attempts to relieve Parkinson’s symptoms) began to fire less often when the saline was given, and with fewer “bursts” of firing – another feature associated with Parkinson’s. The neuron activity decreased at the same time as the symptoms improved: the saline was definitely doing something.We have a lot to learn about what is happening here, Benedetti says, but one thing is clear: the mind can affect the body’s biochemistry. “The relationship between expectation and therapeutic outcome is a wonderful model to understand mind-body interaction,” he says. Researchers now need to identify when and where placebo works. There may be diseases in which it has no effect. There may be a common mechanism in different illnesses. As yet, we just don’t know.2 The horizon problemOUR universe appears to be unfathomably uniform. Look across space from one edge of the visible universe to the other, and you’ll see that the microwave background radiation filling the cosmos is at the same temperature everywhere. That may not seem surprising until you consider that the two edges are nearly 28 billion light years apart and our universe is only 14 billion years old.Nothing can travel faster than the speed of light, so there is no way heat radiation could have travelled between the two horizons to even out the hot and cold spots created in the big bang and leave the thermal equilibrium we see now.This “horizon problem” is a big headache for cosmologists, so big that they have come up with some pretty wild solutions. “Inflation”, for example.You can solve the horizon problem by having the universe expand ultra-fast for a time, just after the big bang, blowing up by a factor of 1050 in 10-33 seconds. But is that just wishful thinking? “Inflation would be an explanation if it occurred,” says University of Cambridge astronomer Martin Rees. The trouble is that no one knows what could have made that happen – but see Inside inflation: after the big bang.So, in effect, inflation solves one mystery only to invoke another. A variation in the speed of light could also solve the horizon problem – but this too is impotent in the face of the question “why?” In scientific terms, the uniform temperature of the background radiation remains an anomaly.“A variation in the speed of light could solve the problem, but this too is impotent in the face of the question ‘why?’”3 Ultra-energetic cosmic raysFOR more than a decade, physicists in Japan have been seeing cosmic rays that should not exist. Cosmic rays are particles – mostly protons but sometimes heavy atomic nuclei – that travel through the universe at close to the speed of light. Some cosmic rays detected on Earth are produced in violent events such as supernovae, but we still don’t know the origins of the highest-energy particles, which are the most energetic particles ever seen in nature. But that’s not the real mystery.As cosmic-ray particles travel through space, they lose energy in collisions with the low-energy photons that pervade the universe, such as those of the cosmic microwave background radiation. Einstein’s special theory of relativity dictates that any cosmic rays reaching Earth from a source outside our galaxy will have suffered so many energy-shedding collisions that their maximum possible energy is 5 × 1019 electronvolts. This is known as the Greisen-Zatsepin-Kuzmin limit.Over the past decade, however, the University of Tokyo’s Akeno Giant Air Shower Array – 111 particle detectors spread out over 100 square kilometres – has detected several cosmic rays above the GZK limit. In theory, they can only have come from within our galaxy, avoiding an energy-sapping journey across the cosmos. However, astronomers can find no source for these cosmic rays in our galaxy. So what is going on?One possibility is that there is something wrong with the Akeno results. Another is that Einstein was wrong. His special theory of relativity says that space is the same in all directions, but what if particles found it easier to move in certain directions? Then the cosmic rays could retain more of their energy, allowing them to beat the GZK limit.Physicists at the Pierre Auger experiment in Mendoza, Argentina, are now working on this problem. Using 1600 detectors spread over 3000 square kilometres, Auger should be able to determine the energies of incoming cosmic rays and shed more light on the Akeno results.Alan Watson, an astronomer at the University of Leeds, UK, and spokesman for the Pierre Auger project, is already convinced there is something worth following up here. “I have no doubts that events above 1020 electronvolts exist. There are sufficient examples to convince me,” he says. The question now is, what are they? How many of these particles are coming in, and what direction are they coming from? Until we get that information, there’s no telling how exotic the true explanation could be.Update: Follow the latest hunt for GZK neutrinos.“One possibility is that there is something wrong with the Akeno results. Another is that Einstein was wrong”4 Belfast homeopathy resultsMADELEINE Ennis, a pharmacologist at Queen’s University, Belfast, was the scourge of homeopathy. She railed against its claims that a chemical remedy could be diluted to the point where a sample was unlikely to contain a single molecule of anything but water, and yet still have a healing effect. Until, that is, she set out to prove once and for all that homeopathy was bunkum.In her most recent paper, Ennis describes how her team looked at the effects of ultra-dilute solutions of histamine on human white blood cells involved in inflammation. These “basophils” release histamine when the cells are under attack. Once released, the histamine stops them releasing any more. The study, replicated in four different labs, found that homeopathic solutions – so dilute that they probably didn’t contain a single histamine molecule – worked just like histamine. Ennis might not be happy with the homeopaths’ claims, but she admits that an effect cannot be ruled out.So how could it happen? Homeopaths prepare their remedies by dissolving things like charcoal, deadly nightshade or spider venom in ethanol, and then diluting this “mother tincture” in water again and again. No matter what the level of dilution, homeopaths claim, the original remedy leaves some kind of imprint on the water molecules. Thus, however dilute the solution becomes, it is still imbued with the properties of the remedy.You can understand why Ennis remains sceptical. And it remains true that no homeopathic remedy has ever been shown to work in a large randomised placebo-controlled clinical trial. But the Belfast study (Inflammation Research, vol 53, p 181) suggests that something is going on. “We are,” Ennis says in her paper, “unable to explain our findings and are reporting them to encourage others to investigate this phenomenon.” If the results turn out to be real, she says, the implications are profound: we may have to rewrite physics and chemistry.5 Dark matterTAKE our best understanding of gravity, apply it to the way galaxies spin, and you’ll quickly see the problem: the galaxies should be falling apart. Galactic matter orbits around a central point because its mutual gravitational attraction creates centripetal forces. But there is not enough mass in the galaxies to produce the observed spin.Vera Rubin, an astronomer working at the Carnegie Institution’s department of terrestrial magnetism in Washington DC, spotted this anomaly in the late 1970s. The best response from physicists was to suggest there is more stuff out there than we can see. The trouble was, nobody could explain what this “dark matter” was.And they still can’t. Although researchers have made many suggestions about what kind of particles might make up dark matter, there is no consensus. It’s an embarrassing hole in our understanding. Astronomical observations suggest that dark matter must make up about 90 per cent of the mass in the universe, yet we are astonishingly ignorant what that 90 per cent is.Maybe we can’t work out what dark matter is because it doesn’t actually exist. That’s certainly the way Rubin would like it to turn out. “If I could have my pick, I would like to learn that Newton’s laws must be modified in order to correctly describe gravitational interactions at large distances,” she says. “That’s more appealing than a universe filled with a new kind of sub-nuclear particle.”Update: Some scientists are trying to create the stuff themselves. See Let there be dark matter.“If the results turn out to be real, the implications are profound. We may have to rewrite physics and chemistry”6 Viking’s methaneJULY 20, 1976. Gilbert Levin is on the edge of his seat. Millions of kilometres away on Mars, the Viking landers have scooped up some soil and mixed it with carbon-14-labelled nutrients. The mission’s scientists have all agreed that if Levin’s instruments on board the landers detect emissions of carbon-14-containing methane from the soil, then there must be life on Mars.Viking reports a positive result. Something is ingesting the nutrients, metabolising them, and then belching out gas laced with carbon-14.So why no party?Because another instrument, designed to identify organic molecules considered essential signs of life, found nothing. Almost all the mission scientists erred on the side of caution and declared Viking’s discovery a false positive. But was it?The arguments continue to rage, but results from NASA’s latest rovers show that the surface of Mars was almost certainly wet in the past and therefore hospitable to life. And there is plenty more evidence where that came from, Levin says. “Every mission to Mars has produced evidence supporting my conclusion. None has contradicted it.”Levin stands by his claim, and he is no longer alone. Joe Miller, a cell biologist at the University of Southern California in Los Angeles, has re-analysed the data and he thinks that the emissions show evidence of a circadian cycle. That is highly suggestive of life.Levin is petitioning ESA and NASA to fly a modified version of his mission to look for “chiral” molecules. These come in left or right-handed versions: they are mirror images of each other. While biological processes tend to produce molecules that favour one chirality over the other, non-living processes create left and right-handed versions in equal numbers. If a future mission to Mars were to find that Martian “metabolism” also prefers one chiral form of a molecule to the other, that would be the best indication yet of life on Mars.Update: Also see our Top 10 controversial pieces of evidence for extraterrestrial life.“Something on Mars is ingesting nutrients, metabolising them and then belching out radioactive methane”7 TetraneutronsFOUR years ago, a particle accelerator in France detected six particles that should not exist (see Ghost in the atom). They are called tetraneutrons: four neutrons that are bound together in a way that defies the laws of physics.Francisco Miguel Marquès and colleagues at the Ganil accelerator in Caen are now gearing up to do it again. If they succeed, these clusters may oblige us to rethink the forces that hold atomic nuclei together.The team fired beryllium nuclei at a small carbon target and analysed the debris that shot into surrounding particle detectors. They expected to see evidence for four separate neutrons hitting their detectors. Instead the Ganil team found just one flash of light in one detector. And the energy of this flash suggested that four neutrons were arriving together at the detector. Of course, their finding could have been an accident: four neutrons might just have arrived in the same place at the same time by coincidence. But that’s ridiculously improbable.Not as improbable as tetraneutrons, some might say, because in the standard model of particle physics tetraneutrons simply can’t exist. According to the Pauli exclusion principle, not even two protons or neutrons in the same system can have identical quantum properties. In fact, the strong nuclear force that would hold them together is tuned in such a way that it can’t even hold two lone neutrons together, let alone four. Marquès and his team were so bemused by their result that they buried the data in a research paper that was ostensibly about the possibility of finding tetraneutrons in the future (Physical Review C, vol 65, p 44006).And there are still more compelling reasons to doubt the existence of tetraneutrons. If you tweak the laws of physics to allow four neutrons to bind together, all kinds of chaos ensues (Journal of Physics G, vol 29, L9). It would mean that the mix of elements formed after the big bang was inconsistent with what we now observe and, even worse, the elements formed would have quickly become far too heavy for the cosmos to cope. “Maybe the universe would have collapsed before it had any chance to expand,” says Natalia Timofeyuk, a theorist at the University of Surrey in Guildford, UK.There are, however, a couple of holes in this reasoning. Established theory does allow the tetraneutron to exist – though only as a ridiculously short-lived particle. “This could be a reason for four neutrons hitting the Ganil detectors simultaneously,” Timofeyuk says. And there is other evidence that supports the idea of matter composed of multiple neutrons: neutron stars. These bodies, which contain an enormous number of bound neutrons, suggest that as yet unexplained forces come into play when neutrons gather en masse.8 The Pioneer anomalyTHIS is a tale of two spacecraft. Pioneer 10 was launched in 1972; Pioneer 11 a year later. By now both craft should be drifting off into deep space with no one watching. However, their trajectories have proved far too fascinating to ignore.That’s because something has been pulling – or pushing – on them, causing them to speed up. The resulting acceleration is tiny, less than a nanometre per second per second. That’s equivalent to just one ten-billionth of the gravity at Earth’s surface, but it is enough to have shifted Pioneer 10 some 400,000 kilometres off track. NASA lost touch with Pioneer 11 in 1995, but up to that point it was experiencing exactly the same deviation as its sister probe. So what is causing it?Nobody knows. Some possible explanations have already been ruled out, including software errors, the solar wind or a fuel leak. If the cause is some gravitational effect, it is not one we know anything about. In fact, physicists are so completely at a loss that some have resorted to linking this mystery with other inexplicable phenomena.Bruce Bassett of the University of Portsmouth, UK, has suggested that the Pioneer conundrum might have something to do with variations in alpha, the fine structure constant. Others have talked about it as arising from dark matter – but since we don’t know what dark matter is, that doesn’t help much either. “This is all so maddeningly intriguing,” says Michael Martin Nieto of the Los Alamos National Laboratory. “We only have proposals, none of which has been demonstrated.”Nieto has called for a new analysis of the early trajectory data from the craft, which he says might yield fresh clues. But to get to the bottom of the problem what scientists really need is a mission designed specifically to test unusual gravitational effects in the outer reaches of the solar system. Such a probe would cost between $300 million and $500 million and could piggyback on a future mission to the outer reaches of the solar system (http://www.arxiv.org/gr-qc/0411077).“An explanation will be found eventually,” Nieto says. “Of course I hope it is due to new physics – how stupendous that would be. But once a physicist starts working on the basis of hope he is heading for a fall.” Disappointing as it may seem, Nieto thinks the explanation for the Pioneer anomaly will eventually be found in some mundane effect, such as an unnoticed source of heat on board the craft.Update: see Computer sleuths try to crack Pioneer anomaly.9 Dark energyIT IS one of the most famous, and most embarrassing, problems in physics. In 1998, astronomers discovered that the universe is expanding at ever faster speeds. It’s an effect still searching for a cause – until then, everyone thought the universe’s expansion was slowing down after the big bang. “Theorists are still floundering around, looking for a sensible explanation,” says cosmologist Katherine Freese of the University of Michigan, Ann Arbor. “We’re all hoping that upcoming observations of supernovae, of clusters of galaxies and so on will give us more clues.”One suggestion is that some property of empty space is responsible – cosmologists call it dark energy. But all attempts to pin it down have fallen woefully short. It’s also possible that Einstein’s theory of general relativity may need to be tweaked when applied to the very largest scales of the universe. “The field is still wide open,” Freese says.Update: see Superconductors inspire quantum test for dark energy, and Dark energy: Seeking the heart of darkness.10 The Kuiper cliffIF YOU travel out to the far edge of the solar system, into the frigid wastes beyond Pluto, you’ll see something strange. Suddenly, after passing through the Kuiper belt, a region of space teeming with icy rocks, there’s nothing.Astronomers call this boundary the Kuiper cliff, because the density of space rocks drops off so steeply. What caused it? The only answer seems to be a 10th planet. We’re not talking about Quaoar or Sedna: this is a massive object, as big as Earth or Mars, that has swept the area clean of debris.The evidence for the existence of “Planet X” is compelling, says Alan Stern, an astronomer at the Southwest Research Institute in Boulder, Colorado. But although calculations show that such a body could account for the Kuiper cliff (Icarus, vol 160, p 32), no one has ever seen this fabled 10th planet.There’s a good reason for that. The Kuiper belt is just too far away for us to get a decent view. We need to get out there and have a look before we can say anything about the region. And that won’t be possible for another decade, at least. NASA’s New Horizons probe, which will head out to Pluto and the Kuiper belt, is scheduled for launch in January 2006. It won’t reach Pluto until 2015, so if you are looking for an explanation of the vast, empty gulf of the Kuiper cliff, watch this space.11 The Wow signalIT WAS 37 seconds long and came from outer space. On 15 August 1977 it caused astronomer Jerry Ehman, then of Ohio State University in Columbus, to scrawl “Wow!” on the printout from Big Ear, Ohio State’s radio telescope in Delaware. And 28 years later no one knows what created the signal. “I am still waiting for a definitive explanation that makes sense,” Ehman says.Coming from the direction of Sagittarius, the pulse of radiation was confined to a narrow range of radio frequencies around 1420 megahertz. This frequency is in a part of the radio spectrum in which all transmissions are prohibited by international agreement. Natural sources of radiation, such as the thermal emissions from planets, usually cover a much broader sweep of frequencies. So what caused it?The nearest star in that direction is 220 light years away. If that is where is came from, it would have had to be a pretty powerful astronomical event – or an advanced alien civilisation using an astonishingly large and powerful transmitter.The fact that hundreds of sweeps over the same patch of sky have found nothing like the Wow signal doesn’t mean it’s not aliens. When you consider the fact that the Big Ear telescope covers only one-millionth of the sky at any time, and an alien transmitter would also likely beam out over the same fraction of sky, the chances of spotting the signal again are remote, to say the least.Others think there must be a mundane explanation. Dan Wertheimer, chief scientist for the SETI@home project, says the Wow signal was almost certainly pollution: radio-frequency interference from Earth-based transmissions. “We’ve seen many signals like this, and these sorts of signals have always turned out to be interference,” he says. The debate continues.Update: see Top 10 controversial pieces of evidence for extraterrestrial life.“It was either a powerful astronomical event – or an advanced alien civilisation beaming out a signal”12 Not-so-constant constantsIN 1997 astronomer John Webb and his team at the University of New South Wales in Sydney analysed the light reaching Earth from distant quasars. On its 12-billion-year journey, the light had passed through interstellar clouds of metals such as iron, nickel and chromium, and the researchers found these atoms had absorbed some of the photons of quasar light – but not the ones they were expecting.If the observations are correct, the only vaguely reasonable explanation is that a constant of physics called the fine structure constant, or alpha, had a different value at the time the light passed through the clouds.But that’s heresy. Alpha is an extremely important constant that determines how light interacts with matter – and it shouldn’t be able to change. Its value depends on, among other things, the charge on the electron, the speed of light and Planck’s constant. Could one of these really have changed?No one in physics wanted to believe the measurements. Webb and his team have been trying for years to find an error in their results. But so far they have failed.Webb’s are not the only results that suggest something is missing from our understanding of alpha. A recent analysis of the only known natural nuclear reactor, which was active nearly 2 billion years ago at what is now Oklo in Gabon, also suggests something about light’s interaction with matter has changed.The ratio of certain radioactive isotopes produced within such a reactor depends on alpha, and so looking at the fission products left behind in the ground at Oklo provides a way to work out the value of the constant at the time of their formation. Using this method, Steve Lamoreaux and his colleagues at the Los Alamos National Laboratory in New Mexico suggest that alpha may have decreased by more than 4 per cent since Oklo started up (Physical Review D, vol 69, p 121701).There are gainsayers who still dispute any change in alpha. Patrick Petitjean, an astronomer at the Institute of Astrophysics in Paris, led a team that analysed quasar light picked up by the Very Large Telescope (VLT) in Chile and found no evidence that alpha has changed. But Webb, who is now looking at the VLT measurements, says that they require a more complex analysis than Petitjean’s team has carried out. Webb’s group is working on that now, and may be in a position to declare the anomaly resolved – or not – later this year.“It’s difficult to say how long it’s going to take,” says team member Michael Murphy of the University of Cambridge. “The more we look at these new data, the more difficulties we see.” But whatever the answer, the work will still be valuable. An analysis of the way light passes through distant molecular clouds will reveal more about how the elements were produced early in the universe’s history.Update: No such thing as a constant constant?13 Cold fusionAFTER 16 years, it’s back. In fact, cold fusion never really went away. Over a 10-year period from 1989, US navy labs ran more than 200 experiments to investigate whether nuclear reactions generating more energy than they consume – supposedly only possible inside stars – can occur at room temperature. Numerous researchers have since pronounced themselves believers.With controllable cold fusion, many of the world’s energy problems would melt away: no wonder the US Department of Energy is interested. In December, after a lengthy review of the evidence, it said it was open to receiving proposals for new cold fusion experiments.That’s quite a turnaround. The DoE’s first report on the subject, published 15 years ago, concluded that the original cold fusion results, produced by Martin Fleischmann and Stanley Pons of the University of Utah and unveiled at a press conference in 1989, were impossible to reproduce, and thus probably false.The basic claim of cold fusion is that dunking palladium electrodes into heavy water – in which oxygen is combined with the hydrogen isotope deuterium – can release a large amount of energy. Placing a voltage across the electrodes supposedly allows deuterium nuclei to move into palladium’s molecular lattice, enabling them to overcome their natural repulsion and fuse together, releasing a blast of energy. The snag is that fusion at room temperature is deemed impossible by every accepted scientific theory.“Cold fusion would make the world’s energy problems melt away. No wonder the Department of Energy is interested”That doesn’t matter, according to David Nagel, an engineer at George Washington University in Washington DC. Superconductors took 40 years to explain, he points out, so there’s no reason to dismiss cold fusion. “The experimental case is bulletproof,” he says. “You can’t make it go away.”Courtesy: 13 things that do not make sense
- Home >
- Catalog >
- Business >
- Report Template >
- Sales Call Report Template >
- Hotel Sales Call Report >
- daily sales report template excel >
- Chemistry 111 - Laboratory Report Form Experiment 2