How to Edit Your Arrangement Of Electrons In Atoms Section 1 The Development Online Easily Than Ever
Follow these steps to get your Arrangement Of Electrons In Atoms Section 1 The Development edited with accuracy and agility:
- Hit the Get Form button on this page.
- You will go to our PDF editor.
- Make some changes to your document, like signing, highlighting, and other tools in the top toolbar.
- Hit the Download button and download your all-set document into you local computer.
We Are Proud of Letting You Edit Arrangement Of Electrons In Atoms Section 1 The Development With a Streamlined Workflow
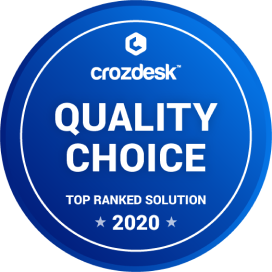
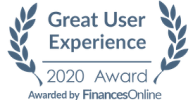
Get Our Best PDF Editor for Arrangement Of Electrons In Atoms Section 1 The Development
Get FormHow to Edit Your Arrangement Of Electrons In Atoms Section 1 The Development Online
If you need to sign a document, you may need to add text, fill out the date, and do other editing. CocoDoc makes it very easy to edit your form with just a few clicks. Let's see how this works.
- Hit the Get Form button on this page.
- You will go to CocoDoc online PDF editor webpage.
- When the editor appears, click the tool icon in the top toolbar to edit your form, like highlighting and erasing.
- To add date, click the Date icon, hold and drag the generated date to the target place.
- Change the default date by changing the default to another date in the box.
- Click OK to save your edits and click the Download button when you finish editing.
How to Edit Text for Your Arrangement Of Electrons In Atoms Section 1 The Development with Adobe DC on Windows
Adobe DC on Windows is a useful tool to edit your file on a PC. This is especially useful when you prefer to do work about file edit offline. So, let'get started.
- Click the Adobe DC app on Windows.
- Find and click the Edit PDF tool.
- Click the Select a File button and select a file from you computer.
- Click a text box to modify the text font, size, and other formats.
- Select File > Save or File > Save As to confirm the edit to your Arrangement Of Electrons In Atoms Section 1 The Development.
How to Edit Your Arrangement Of Electrons In Atoms Section 1 The Development With Adobe Dc on Mac
- Select a file on you computer and Open it with the Adobe DC for Mac.
- Navigate to and click Edit PDF from the right position.
- Edit your form as needed by selecting the tool from the top toolbar.
- Click the Fill & Sign tool and select the Sign icon in the top toolbar to customize your signature in different ways.
- Select File > Save to save the changed file.
How to Edit your Arrangement Of Electrons In Atoms Section 1 The Development from G Suite with CocoDoc
Like using G Suite for your work to complete a form? You can integrate your PDF editing work in Google Drive with CocoDoc, so you can fill out your PDF without Leaving The Platform.
- Go to Google Workspace Marketplace, search and install CocoDoc for Google Drive add-on.
- Go to the Drive, find and right click the form and select Open With.
- Select the CocoDoc PDF option, and allow your Google account to integrate into CocoDoc in the popup windows.
- Choose the PDF Editor option to open the CocoDoc PDF editor.
- Click the tool in the top toolbar to edit your Arrangement Of Electrons In Atoms Section 1 The Development on the specified place, like signing and adding text.
- Click the Download button to save your form.
PDF Editor FAQ
What is the biggest mind blow ever?
This is absolutely new research in the field of Electronics. I am damn sure that most of you have never heard about something like this before. I have tried to explain it in most easy way. Hopefully you all will be able to understand.We all use Smartphones in our day to day life. And we are witnessing that in last few years the thickness of mobile phones are decreasing. Thanks to VLSI for it. But researchers at Hong Kong have developed something new. Which will help in decreasing the thickness of smartphones more & that’s too even with out introducing VLSI in it.Have you ever heard about the battery which acts as a power supply and also as a full color display? No, right?So let’s know about it.We all know that in our mobile phones batteries are only used for supplying power to the electronics component, so that they can work.Scientists have developed a semi transparent arrangement of cathode & anode with a transparent micro battery. This battery works like normal Li-ion battery . This battery is made of mixture of gelatin & fluorescent quantum dots. Under the effect of light, fluorescent components emits light (Basically three primary colours Red, Green & Blue). And this is how the concept of battery in screen can be converted into reality.It’s color property is more because of fluorescent quantum dots. Quantum dots are tiny particles made of semiconductor material. It’s diameter is usually in the range of nanometer order. Quantum dots have discrete, quantized energy levels which relate them more closely to atoms. As the crystal size decreases the energy difference between valence band and conduction band Increases hence more energy is needed to excite the dot & that’s why more energy get released when atom returns to it ground state. And because of it color of emitted light get shifted in batteries.If we talk about the structure of these batteries than it have sandwich like structure. On the transparent surface of polymer the positive and negative electrodes are deposited.So in future this concept will surely get commercialized. And our smartphone will get more thinner. Batteries will act as a display and power supply source both.Hope it helps!!You can share your doubts in the comment section or can message me on my twitter handle Twitter or on my Instagram ID Instagram .Satyam SinghCheers!!
Who was/is the most badass scientist?
Who’s the most badass scientist ever? How about someone who mindf#cked “the greatest living scientist” of his time…… as well as the most powerful political force of his time (pre-WW2)…… as well as the ideas that formed the very foundation of physics itself (and by extension the foundation of our industrial civilization)?Someone who made a key contribution in the creation of the first atomic bomb, and also foretold, in stunning precision and long before any political leader realized it, of the unprecedented promise of global peace and international cooperation that our ability to harness nuclear energy brings, and also the unprecedented threat it poses to our existence if nations don’t come together.And someone who didn’t just stop at theorizing or philosophizing the idea that nations that have fought each other for centuries could coalesce for a greater good but actually made it happen, when he led an effort that brought twelve European countries together to create the first truly international research organization, which today operates the world’s pre-eminent particle physics laboratory, and also is the birthplace of a little thing called The World Wide Web:One of the greatest theoretical physicists of all time, a philosopher who pondered some of the most inscrutable aspects of the very nature of reality itself, but also a prescient realist and a connoisseur of international politics who could influence leaders of nations to do good! Almost a mythical hero, from the land of Hans Christian Andersen, his name was Niels Henrik David Bohr.Badassery Exhibit A: At age 20, refined Lord Rayleigh’s theory and methods of measuring surface tension of liquids. To quote Wikipedia:In 1905, a gold medal competition was sponsored by the Royal Danish Academy of Sciences and Letters to investigate a method for measuring the surface tension of liquids that had been proposed by Lord Rayleigh in 1879. This involved measuring the frequency of oscillation of the radius of a water jet. Bohr conducted a series of experiments using his father's laboratory in the university; the university itself had no physics laboratory. To complete his experiments, he had to make his own glassware, creating test tubes with the required elliptical cross-sections. He went beyond the original task, incorporating improvements into both Rayleigh's theory and his method, by taking into account the viscosity of the water, and by working with finite amplitudes instead of just infinitesimal ones. His essay, which he submitted at the last minute, won the prize. He later submitted an improved version of the paper to the Royal Society in London for publication in the Philosophical Transactions of the Royal Society.Badassery Exhibit B: Gave birth to a new physics. In the 1880s, physicists Johann Balmer and Johannes Rydberg discovered that the wavelengths of the emitted light in the spectrum of hydrogen follow a simple pattern:where R(H) is a constant, called the Rydberg constant for hydrogen (approximately 10967758 m^−1), and n1, n2 are just natural numbers (n2 > n1).For decades, no one could explain why this happens. Then, in 1911, Ernest Rutherford put forth his solar-system-like model of the atom: negatively charged electrons orbiting a positively charged nucleus. The main issue with this model was that it violates classical electrodynamics, which says that in such a system the electron would gradually lose its energy and spiral into the nucleus, thus making the whole system unstable. At this juncture, in 1913, Bohr had the seminal insight that the fixed wavelength values of hydrogen spectrum found by Balmer and Rydberg can be simply explained as energy emitted by electrons jumping from a higher-energy orbit to a lower-energy orbit. Applying Max Planck’s quantum theory to define the energy of the electron, he was able to calculate the value of the Rydberg constant for hydrogen which matched the experimentally observed value. Thus Bohr proposed a refined version of Rutherford’s model which stated that even though electrons are negatively charged and they do orbit the positively charged nucleus, they do so in specific orbits of quantized energy levels in which they do not gradually lose energy, but only do so when they jump from a higher quantum level to a lower quantum level, by emitting radiation with energy equaling the difference of energy between the levels.The implication of such a finding was momentous! It meant that classical mechanics, which had been successfully describing the motion of heavenly bodies (mostly) to the movement of most everyday objects, is woefully inadequate in explaining processes at the subatomic level, and must be superseded by a new physics — which is what we today call the Quantum Mechanics.Badassery Exhibit C: Transformed our understanding of the periodic table of elements. The German chemist Johann Döbereiner made the first known attempt to classify elements on the basis of similarity in their physicochemical properties, when he grouped 12 elements into 4 “triads” (chlorine-bromine-iodine, lithium-sodium-potassium, sulfur-selenium-tellurium and calcium-strontium-barium) in the 1820s. (It’s interesting to note that such triads actually do exist in the modern periodic table taking only naturally occurring elements into account, e.g. zinc-cadmium-mercury, copper-silver-gold, etc.!!) He also noted that the atomic weight of the middle element in a triad was somehow very close to the mean of those of the other two! Then, as a lot more elements got discovered and their atomic weights measured, multiple chemists started noticing (in the 1860s) that if ordered by increasing atomic weight, elements display similar properties at regular intervals, and not just in groups of threes! The most notable among them, Dmitri Mendeleev, was able to classify all known elements into 8 Groups, in what he called Periodicheskaya Tablitsa Elementov, Russia’s single biggest contribution to science!But of course, there were problems with this arrangement, for example, it was found that iodine is actually lighter than tellurium, even though it has to be placed after tellurium in order to group them with similar elements! Also, Mendeleev placed iron-ruthenium-osmium in the same group with copper-silver-gold (Group VIII) even though there are significant differences in their properties – in fact, he got a bit confused and suggested that copper-silver-gold might belong to Group I instead! In the end, the discovery of isotopes was this periodic table’s final death knell because it meant that two atoms of the same element can have different weights (and two atoms of different elements can have the same weight, for example, an atom of carbon can have the same weight as an atom of nitrogen), hence the atomic weight is not at all something that defines an element in the first place!At this juncture, the team of Bohr, Rutherford, Henry Moseley and others at Cambridge made some major discoveries that unwrapped many of the remaining mysteries of the atomic constitution, including the discovery of the proton, the concept of the Atomic Number as the unique identifier of an element as opposed to the atomic weight, and the fact that the atomic number is nothing but the number of protons in the atom, which is equal to the nuclear charge of the atom, which is then equal to the number of electrons in the atom (in neutral state). These discoveries made it clear that instead of atomic weight, the elements should be arranged by the atomic number in the periodic table, which did resolve questions such as how tellurium precedes iodine, etc. but couldn’t offer a solution for the other issues, let alone explain what really causes the periodicity…At this time, Bohr, working with Arnold Sommerfeld, Wolfgang Pauli and others, turned his attention to using quantum physics to understand how electrons are distributed in different orbitals in atoms of different elements, and formulated what became known as the Aufbauprinzip or “building-up principle”. They realized that the electrons don’t just exist in the primary orbitals but in various sub-shells within the orbitals, which they called s, p, d and f, and defined the order of increasing energy of these sub-shells, which determines (along with Pauli’s Exclusion Principle and other laws), when we move from one element to the next, where the new electron is added.Using these principles, they worked out the electron configurations of various elements, which looked like this:The pattern was unmissable! Bohr realized that the “similar” elements in the periodic table are similar simply because they have the same electron configurations in the outermost sub-shells of their atoms! For example, alkali metals like lithium, sodium, etc. always end with ns1, alkaline earth metals (beryllium, magnesium, calcium, etc.) with ns2, boron-aluminum-gallium-… with np1, carbon-silicon-germanium-… with np2, and so on. Although there were some exceptions (mainly the “transition metals” like chromium, copper, and the series from niobium to silver), based on overall similarities, all elements could now be classified into 18 groups (32 if including lanthanides and actinides)… and thus the modern periodic table was born.This fact that an element's physicochemical (macro) properties are nothing but manifestations of the arrangement of electrons in its atom (micro) is one of the most supremely important discoveries in the history of science, the ultimate confluence of physics and chemistry, and a triumph of quantum theory!! If it were to happen today, Bohr would immediately win his second Nobel prize, possibly in chemistry, but in an age where new elements and isotopes were being discovered and created almost on daily basis, he missed out!!!Badassery Exhibit D: Stood up to Einstein to defend own theory, and prevailed. Between 1925 and ‘27, Bohr, along with Werner Heisenberg, Max Born and others, developed what came to be known as the Copenhagen Interpretation of quantum mechanics, centered around Heisenberg’s Uncertainty Principle, Born’s Born Rule of the probabilistic wave function, and Bohr’s Complementarity Principle of wave-particle duality. This interpretation essentially proposed that:physical systems generally do not have definite properties prior to being measured, and quantum mechanics can only predict the probability distribution of a given measurement's possible results. The act of measurement affects the system, causing the set of probabilities to reduce to only one of the possible values immediately after the measurement.While many physicists were in a state of dismay and awe at the mathematical ingenuity of this theory, Einstein came out with strong disagreement. He simply refused to accept that physical realities could be just statistical probabilities and couldn’t be measured beyond a certain degree of accuracy, summed up in his famous remark “God doesn’t throw dice”. The Solvay Conference of 1927 brought this bitter conflict out in the open.Over dinner, during after-dinner discussions, and at breakfast, Einstein debated with Bohr and his followers on the question whether quantum mechanics in its present form could be called complete. Einstein illustrated his points with increasingly clever thought experiments intended to prove that position and momentum could in principle be simultaneously known to arbitrary precision. For example, one of his thought experiments involved sending a beam of electrons through a shuttered screen, recording the positions of the electrons as they struck a photographic screen. Bohr and his allies would always be able to counter Einstein's proposal, usually by the end of the same day… By 1928, the consensus was that Einstein had lost the debate, and even his closest allies… conceded that quantum mechanics appeared to be complete.But 3 years later, at the next Solvay Conference, Einstein came prepared for a smackdown…He proposed another thought experiment, known as Einstein’s Box.Einstein considers a box containing electromagnetic radiation and a clock which controls the opening of a shutter which covers a hole made in one of the walls of the box. The shutter uncovers the hole for a time Δt which can be chosen arbitrarily. During the opening, we are to suppose that a photon, from among those inside the box, escapes through the hole. In this way a wave of limited spatial extension has been created, following the explanation given above. In order to challenge the indeterminacy relation between time and energy, it is necessary to find a way to determine with adequate precision the energy that the photon has brought with it. At this point, Einstein turns to his celebrated relation between mass and energy of special relativity: E = mc^2. From this it follows that knowledge of the mass of an object provides a precise indication about its energy. The argument is therefore very simple: if one weighs the box before and after the opening of the shutter and if a certain amount of energy has escaped from the box, the box will be lighter. The variation in mass multiplied by c^2 will provide precise knowledge of the energy emitted. Moreover, the clock will indicate the precise time at which the event of the particle's emission took place. Since, in principle, the mass of the box can be determined to an arbitrary degree of accuracy, the energy emitted can be determined with a precision ΔE as accurate as one desires. Therefore, the product ΔEΔt can be rendered less than what is implied by the principle of indeterminacy.This challenge shell-shocked Bohr, “who, at first, could not think of a solution. For the entire evening he was extremely agitated, and he continued passing from one scientist to another, seeking to persuade them that it could not be the case, that it would have been the end of physics if Einstein were right; but he couldn't come up with any way to resolve the paradox”, remembers fellow attendee Leon Rosenfeld, “I will never forget the image of the two antagonists as they left the club: Einstein, with his tall and commanding figure, who walked tranquilly, with a mildly ironic smile, and Bohr who trotted along beside him, full of excitement.” (Below is an actual photo of this!)However, the next morning, Bohr dealt the knockout punch, using the relativistic time dilation principles of Einstein himself (also called Gravitational Redshift):1. After emitting a photon, the loss of weight causes the box to rise in the gravitational field.2. The observer returns the box to its original height by adding weights until the pointer points to its initial position. It takes a certain amount of time t for the observer to perform this procedure. How long it takes depends on the strength of the spring and on how well-damped the system is. If undamped, the box will bounce up and down forever. If over-damped, the box will return to its original position sluggishly.3. The longer that the observer allows the damped spring-mass system to settle, the closer the pointer will reach its equilibrium position. At some point, the observer will conclude that his setting of the pointer to its initial position is within an allowable tolerance. There will be some residual error Δq in returning the pointer to its initial position. Correspondingly, there will be some residual error Δm in the weight measurement.4. Adding the weights imparts a momentum p to the box which can be measured with an accuracy Δp delimited by ΔpΔq ≈ h. It is clear that Δp < gtΔm where g is the gravitational constant. Plugging in yields gtΔmΔq > h.5. General relativity informs us that while the box has been at a height different than its original height, it has been ticking at a rate different than its original rate. The red shift formula informs us that there will be an uncertainty Δt = c^-2gtΔq in the determination of t0, the emission time of the photon.6. Hence, c^2ΔmΔt = ΔEΔt > h. The accuracy with which the energy of the photon is measured restricts the precision with which its moment of emission can be measured, following the Heisenberg uncertainty principle.Einstein never attacked the basics of the Bohr school of quantum mechanics again!Badassery Exhibit E: Outmaneuvered the Nazis over and over. Unlike many Jewish academics who left Europe in the wake of Nazi aggression, Bohr stood his ground till the very end, and put considerable effort to get a number of Jewish scientists out of Germany and Nazi-occupied parts of Europe to the relative safety of his Institute For Theoretical Physics at Copenhagen, and later used his connections with the Danish resistance to dispatch them to Sweden and other safe countries. Those that he helped include Guido Beck, Felix Bloch, James Franck, George de Hevesy, Otto Frisch, Hilde Levi, Lise Meitner, George Placzek, Eugene Rabinowitch, Stefan Rozental, Erich Ernst Schneider, Edward Teller, Arthur von Hippel and Victor Weisskopf.Now, one of these guys, James Franck, had kept his Nobel prize medal with Bohr for safekeeping, and so had the non-Jewish but vehemently anti-Nazi physicist Max von Laue. As German tanks finally rolled into Copenhagen (April 1940), keeping the two medals out of German hand was Bohr’s primary concern (he had donated his own medal to an auction for the benefit of a fund helping the war-affected Finns a few weeks before this). And just losing the medals was not the only worry! In Hitler’s Germany it was almost a capital offense to send gold out of the country. Since the names of the laureates were engraved on the medals, their discovery by the invading forces would have had very serious consequences for Bohr (and also for von Laue who was in Germany). Bohr knew that the Germans knew that certain Nobel laureates had kept their medals with Bohr and were determined to find them, hence he decided he couldn’t risk the medals being discovered intact. With no other alternative and the Gestapo almost at doorstep, he, with help of George de Hevesy, decided to science his way out!They went to the chemistry lab, grabbed the supply of hydrochloric acid and nitric acid and mixed a deadly cocktail that was first mentioned by Arab alchemists a thousand years ago, called Aqua Regia — “regal water” — the only known liquid that can dissolve gold! Hurriedly dissolving the medals in the acid, they kept the flask in an ordinary place in the lab, amidst dozens of similar-looking flasks containing sundry chemical solutions! The Germans came, and ransacked the entire Institute for years looking for the medals, as Bohr stayed put at Copenhagen.The reason he could stay there, however, was because the German occupation of Denmark was by far the most peaceful of all Nazi-occupied countries. German propaganda even referred to Denmark as the "model protectorate". The king retained his throne and the Danish government continued to function…… and the Danes managed to exact an extraordinary price for this cooperation:Danish officials repeatedly insisted to the German occupation authorities that there was no "Jewish problem" in Denmark. The Germans recognized that discussion of the "Jewish question" in Denmark was a possibly explosive issue… the German Reich relied substantially upon Danish agriculture, which supplied meat and butter to 3.6 million Germans in 1942. As a result… even ideologically committed Nazis… followed a strategy of avoiding and deferring any discussion of Denmark's Jews.In mid-1943, however, having seen German defeats in Russia and North Africa, Danes began to show their displeasure with the occupation, in form of nationwide strikes and increased sabotage activities by the Danish resistance. Finally the German authorities gave the Danish government an ultimatum on August 28, demanding a ban on strikes, a curfew, and the punishment of sabotage with the death penalty. The Danish government rejected this demand and resigned the next day, resulting in direct administration of Denmark by the German authorities, who immediately drew up plans to start rounding up the Jews from early October. In response, the Jews started going into hiding, but that would not save them for long. The best way out was to get Sweden to offer them asylum till the end of the war but the Swedes refused to officially announce anything, in order to avoid increased confrontation with the Germans.In this situation, Bohr decided to throw his weight behind the issue. He contacted Danish resistance and planned an escape to Sweden. (Somehow the Nazis got wind of it and went after him at his home. As they entered through the front door, the 58-year-old Bohr ran out the back, with Danish resistance laying down cover fire, allowing him to slip out and get on a fishing boat!) As he reached Sweden, the government representatives told him that an aircraft was waiting to take him to United States where his expertise was needed in some “special government projects”. Bohr responded that he would not be going anywhere until the fate of his country’s Jews was secure, and requested an audience with the King to discuss the matter. King Gustaf V granted the audience on the afternoon of October 2, it’s said, after a persuasive call from Greta Garbo, who knew Bohr! A few hours later, the Swedish radio broadcast that Sweden was ready to receive the Jews!A massive rescue operation then began to smuggle the 7800-strong Danish Jewry across the Øresund, through the prying eyes of German patrol, and ultimately an estimated 7220 (plus 686 non-Jewish spouses) were able to reach the Swedish shores, making Danish Jews, out of the Jewish populations of all Nazi-occupied countries, the least affected by the Holocaust.Having secured the safety of the Danish Jews, Bohr finally decided to leave for the United States, via Britain. Stockholm was crawling with German agents, and there was a credible threat that he could be assassinated. To fly him to Britain over the dangerous North Sea, the British sent an unarmed Mosquito bomber, a light, fast aircraft that could fly high enough to avoid the German anti-aircraft batteries. At the opportune hour, Bohr donned a flight suit, strapped on a parachute, took delivery of the flight helmet with built-in earphones for communication with the cockpit, and lay on his back in the bomb bay. The pilot also supplied him with a stick of flares — in case the plane was hit and couldn’t make it to British mainland, the pilot was to open the bomb bay doors and drop Bohr into the sea; a following sea plane was then to pick him up… if he survived the fall using his parachute and was able to signal his location using the flares!Ultimately, although those scenarios didn’t unfold, something did go wrong during the flight — the helmet given to Bohr turned out to be quite small, and he couldn’t wear it (“The Royal Air Force was not used to such great heads as Bohr’s”, quips Robert Oppenheimer!), hence when the plane reached high altitude and the pilot instructed Bohr to turn on the oxygen supply, he didn’t hear anything, and soon passed out due to lack of oxygen. Thankfully the pilot realized what had happened, and descended to a lower altitude, saving Bohr’s life!By the way, what happened to the two Nobel prize medals dissolved in aqua regia at Bohr’s Institute at Copenhagen? After the war, as Bohr and de Hevesy returned to the institute, they found that flask exactly as they had left it! They carefully extracted the gold from the solution and sent it to the Swedish Academy, with a letter explaining the situation — the Nobel Foundation then recast the medals from the same gold and re-presented them to Franck and von Laue!!!!!Badassery Exhibit F: Saved America’s plutonium bomb project. Ok, “saved” may be a slight exaggeration but he did make a critical contribution without which the project would get delayed by many months, consequently delaying the Japanese surrender and causing countless more loss of life. The scientists at the Manhattan project were working on two types of bombs. One was straightforward: it consisted of two sub-critical masses of U-235, one containing a neutron source. When the bomb is to be detonated, one of the masses will be inserted into the other, resulting in criticality, and thence the explosive chain reaction. The other approach was to build an implosion device, in which a number of high-explosive charges will go off at the same time imploding a hollow sphere of plutonium (with neutron source at center) to a critical mass. There were serious challenges with both approaches. Even though the uranium bomb was straightforward, the supply of U-235, which is present in uranium in very small amounts, was not adequate for mass production. On the other hand, plutonium was being produced in the nuclear reactors abundantly but building a properly functioning implosion device turned out to be a formidable engineering challenge, the most complex aspect of which was designing that neutron source, called the “modulated neutron initiator”: a device that would produce a burst of neutrons to initiate the chain reaction at the optimal moment when the configuration is “prompt critical”! “The device remained a stubborn puzzle”, noted project director Oppenheimer, which he needed Bohr to crack. Finally in February 1945, under Bohr’s guidance the team succeeded in building the device, and within 5 months the first plutonium bomb was successfully tested.Badassery Exhibit G: Realized the far-reaching consequences of the atomic bomb, sought to bring the Russians in confidence “before there could be any question of use of atomic weapons” and proposed formation of an international body governing the use of nuclear energy, before anyone else. Whereas most scientists at Manhattan project regarded building the bomb as dealing with an existential threat i.e. the (possible) Nazi-made atomic bomb, one wonders if Bohr had some insider information that the German nuclear bomb project was a dud, which made him worry less about the immediate use of the bomb and ponder more about the future, in which “means will be found to simplify the methods of production of the active substances and intensify their effects to an extent which may permit any nation… to command powers of destruction surpassing all previous imagination… Quite apart from the role atomic weapons might come to play in the war [meaning WW2], it was clear that permanent grave dangers to world security would ensue unless measures to prevent abuse of the new formidable means of destruction could be universally agreed upon and carried out.”(Or perhaps, as an ardent believer in the philosophy of complementarity, Bohr could see more clearly than others how the promise of a lasting global peace and international concourse and even cleaner environment went hand in hand with the specter of utter ruin, in the context of unlocking the limitless energy of the atom.)Furthermore, whereas no top-level scientist or official in Britain/America could imagine that the Russians could get the bomb any time soon, Bohr happened to know better.In April 1944, he received a letter from [Russian Nuclear Physicist] Peter Kapitza, written some months before when Bohr was in Sweden, inviting him to come to the Soviet Union. The letter convinced Bohr that the Soviets were aware of the Anglo-American project, and would strive to catch up. He sent Kapitza a non-committal response, which he showed to the authorities in Britain before posting. Bohr met Churchill on 16 May 1944, but found that "we did not speak the same language". Churchill disagreed with the idea of openness towards the Russians to the point that he wrote in a letter: "It seems to me Bohr ought to be confined or at any rate made to see that he is very near the edge of mortal crimes."In America, however, Oppenheimer did see Bohr’s point, and suggested him to speak to POTUS!In that meeting (August 1944), Bohr submitted to Roosevelt what Churchill had failed to grasp: “the very necessity of a concerted effort to forestall such ominous threats to civilization would offer quite unique opportunities to bridge international divergences... early consultations between the nations allied in the war about the best ways jointly to obtain future security might contribute decisively to that atmosphere of mutual confidence which would be essential for co-operation on the many other matters of common concern”.Roosevelt was more understanding than Churchill and advised Bohr to return to Britain to get their approval, as he could not take this decision on his own. However, in 3 weeks’ time, Roosevelt and Churchill had a meeting, where they discussed Bohr’s proposals, and unfortunately, Churchill succeeded in convincing Roosevelt to outright reject them (further noting: “enquiries should be made regarding the activities of Professor Bohr and steps taken to ensure that he is responsible for no leakage of information, particularly to the Russians”). As a direct result of this, for next 7 decades (and likely to continue for untold more decades to come) America and Russia spent trillions of Dollars building, safeguarding, modernizing and also partially dismantling an arsenal of over a hundred thousand nuclear warheads, some of which are kept on hair-trigger alert, ready to snuff out humanity in a nuclear winter.Even after being snubbed by short-sighted, xenophobic, jingoistic politicians, Bohr kept trying. Between 1945 and ’48, he wrote several memos to US statesmen, and even met the Secretary of State, but all his warnings/suggestions were summarily ignored.And then… everything changed.On September 1,1949, the US Air Force Office of Atomic Energy found the first evidence of a Soviet nuclear test (actually conducted, in utter secrecy, 3 days earlier), sending shockwaves (figuratively speaking!) through Truman administration, from which they took 3 weeks to recover in order to announce the news to public! With the people in power finally forced to pay attention, Bohr decided to put together all his thoughts about how to deal with this most extraordinary crisis in the history of our species, in an “Open Letter”, presented to the United Nations on June 9, 1950. The letter was not just “open” in name but also in spirit, and substance! In it, he candidly talked about his own failure to convince the British/American leaders about the dangerous consequences of them unilaterally developing nuclear weapons and then using them in war without sharing any information with the Soviets and greater international community, and also alleged that this discretion was a key reason behind the deepening divide between the wartime allies despite conciliatory efforts of the UN:When the war ended and the great menaces of oppression to so many peoples had disappeared, an immense relief was felt all over the world. Nevertheless, the political situation was fraught with ominous foreboding. Divergences in outlook between the victorious nations inevitably aggravated controversial matters arising in connection with peace settlements. Contrary to the hopes for future fruitful co-operation, expressed from all sides and embodied in the Charter of the United Nations, the lack of mutual confidence soon became evident. The creation of new barriers, restricting the free flow of information between countries, further increased distrust and anxiety.He asserted that for our civilization to endure, and progress, in the nuclear age, nations must embrace a culture of “mutual openness” and “genuine cooperation”: “barriers between nations which hitherto were thought necessary for the defence of national interests would now… stand in the way of common security”. He advocated building “an open world”, where scientific information is exchanged freely between nations, noting that this “may appear utopian” but “such a course should be in the deepest interest of all nations, irrespective of differences in social and economic organization” because “the progress of science and technology has tied the fate of all nations inseparably together”.(It’s interesting to note that this letter coincided with the Stockholm Appeal initiative, which called for an absolute ban on nuclear weapon, and went viral throughout the West but ultimately failed to achieve anything substantial. Bohr was pragmatic enough to avoid any such appeal in his letter!)Lastly, he prescribed the creation of an international organization for monitoring and controlling the nuclear activities of every nation:Any arrangement which can offer safety against secret preparations for the mastery of the new means of destruction would… demand extraordinary measures. In fact, not only would universal access to full information about scientific discoveries be necessary, but every major technical enterprise, industrial as well as military, would have to be open to international control…. Detailed proposals for the establishment of an effective control would have to be worked out with the assistance of scientists and technologists appointed by the governments concerned, and a standing expert committee, related to an international security organization, might be charged with keeping account of new scientific and technical developments and with recommending appropriate adjustments of the control measures.7 years later, the world finally had to create this organization:The same year (1957), Bohr was honored with the first ever Atoms For Peace award.Badassery Exhibit H: Co-founded CERN. As Bohr got increasingly frustrated with his repeated appeals to enforce collaboration between Anglo-American and Soviet nuclear scientists falling to deaf ears, in the end he decided to do something on his own, in a place where he had a little more influence: Western Europe. Working with a few nuclear physicists still left there, he mobilized a massive effort to convince European countries to join together to build a cutting-edge research center that could help European nuclear physics rise again to its pre-war glory — an institute built along the lines of the national laboratories in the US, designed to undertake Big Science projects beyond the resources of any one country alone.In June 1950, the same month he submitted his open letter to the UN, a resolution was tabled at UNESCO to "assist and encourage the formation of regional research laboratories in order to increase international scientific collaboration". A year and half later, the first resolution concerning the establishment of a European Council for Nuclear Research (Conseil Européen pour la Recherche Nucléaire) was adopted. On September 29, 1954, following ratification by France and Germany (with Belgium, Denmark, Greece, Italy, Netherlands, Norway, Sweden, Switzerland, UK and Yugoslavia to follow), CERN was born. (Bohr wanted the center to be set up in Copenhagen but in view of the future needs of the organization to scale up, agreed on the location on France-Switzerland border, in the suburbs of Geneva. The CERN Theory Group, which Bohr headed, was based in Copenhagen until their new accommodation in Geneva was ready, in 1957.)As nuclear physics research steadily declined in the US post the seventies, CERN gradually became its de facto global headquarters, achieving major breakthroughs like the discovery of neutral currents and W and Z bosons, creation of antihydrogen, and detection of the Higgs boson, the ultimate holy grail of particle physics, vindicating Bohr’s belief that in science, the best way to go faster and farther is to go together.I rest my case.Upvote if you agree!Sources:Niels Bohr - Wikipediahttps://www.gutenberg.org/files/47464/47464-pdf.pdfHistory of the periodic table - WikipediaAufbau principle - WikipediaBohr–Einstein debates - WikipediaEinstein's thought experiments - WikipediaA unique gold medalNiels Bohr’s Flight From the Nazis Was a Science DramaRescue of the Danish Jews - WikipediaBohr Letter to UN
How does a multiple bond behave as a single bond?
Chemical bonding, any of the interactions that account for the association of atoms into molecules, ions, crystals, and other stable species that make up the familiar substances of the everyday world. When atoms approach one another, their nuclei and electrons interact and tend to distribute themselves in space in such a way that the total energy is lower than it would be in any alternative arrangement. If the total energy of a group of atoms is lower than the sum of the energies of the component atoms, they then bond together and the energy lowering is the bonding energy.crystal bondingDifferent types of bonding in crystals.Encyclopædia Britannica, Inc.The ideas that helped to establish the nature of chemical bonding came to fruition during the early 20th century, after the electron had been discovered and quantum mechanics had provided a language for the description of the behaviour of electrons in atoms. However, even though chemists need quantummechanics to attain a detailed quantitative understanding of bond formation, much of their pragmaticunderstanding of bonds is expressed in simple intuitive models. These models treat bonds as primarily of two kinds—namely, ionic and covalent. The type of bond that is most likely to occur between two atoms can be predicted on the basis of the location of the elements in the periodic table, and to some extent the properties of the substances so formed can be related to the type of bonding.A key concept in a discussion of chemical bonding is that of the molecule. Molecules are the smallest units of compounds that can exist. One feature of molecules that can be predicted with reasonable success is their shape. Molecular shapes are of considerable importance for understanding the reactions that compounds can undergo, and so the link between chemical bonding and chemical reactivity is discussed briefly in this article.Although simple models of bonding are useful as rules of thumb for rationalizing the existence of compounds and the physical and chemical properties and structures of molecules, they need to be justified by appealing to more-sophisticated descriptions of bonding. Moreover, there are some aspects of molecular structure that are beyond the scope of the simple theories. To achieve this insight, it is necessary to resort to a fully quantum mechanical description. In practice, these descriptions entail heavy reliance on computers. Such numerical approaches to the chemical bond provide important information about bonding.This article begins by describing the historical evolution of the current understanding of chemical bonding and then discusses how modern theories of the formation of chemical bonds have emerged and developed into a powerful description of the structure of matter. After the historical introduction, qualitative models of bonding are discussed, with particular attention given to the formation of ionic and covalent bonds and the correlation of the latter with molecular shapes. The more-sophisticated quantum mechanical approaches to bond formation are then described, followed by a survey of a number of special cases that raise interesting problems or lead to important insights.For a detailed discussion of the structure and properties of atoms, see atom. Chemical compounds are surveyed in the article chemical compound, and the elements are described in the article chemical element.Historical reviewAtomic structure and bondingBonds between atomsIt has been shown that, for reasons related to the energy requirements for electron removal or addition, only the electrons invalence shells play a significant role in the formation of bonds between atoms. Henceforth this article will concentrate on these electrons alone.Lewis introduced the conventions of representing valence electrons by dots arranged around the chemical symbol of the element, as in H· and Na·, and of discussing bond formation as the transfer of dots from one symbol to another. This seemingly simplistic device turns out to be very useful for establishing the characteristics of chemical bonds and will be examined in this section.The formation ofionic bondsLewis formulation of an ionic bondIn Lewis terms, the formation of an ionic bond stems from the transfer ofelectrons from one atom to another. When such a transfer occurs, all the valence electrons on the more electropositive element (from one of the first three groups on the left in the periodic table) are removed to expose the core of the atom. The electrons so released are accepted into the empty orbitals of the valence shell of the more electronegativeatom (typically from the groups immediately to the left of the noble gases); the valence shell is thereby filled. Thus, the formation of the ionic compoundsodium chloride can be represented by the following process:The formation of aluminum oxide (alumina) involves selecting enough aluminum and oxygen atoms to ensure that all the electrons released by the aluminum atoms (three from each one) are accommodated by oxygen atoms (each of which can accept two electrons):(The numbers of atoms required to balance the electrons donated and accepted is indicated by the chemical formula Al2O3for aluminum oxide.)That the transfer of electrons represented by these diagrams leads to a lowering of energy can be checked by assessing the energies associated with them. There is more to the process than a straightforward consideration of ionization energyand electron affinity. The ionization energy of sodium is larger than the electron affinity of chlorine, so energy is required to remove an electron from a sodium atom and attach it to a chlorine atom. That is, at first sight it appears that the total energy of a Na+ion and a Cl−ion is greater than that of a sodium atom and a chlorine atom. If that were the case, then it would be hard to understand how sodium chloride could be a stable species relative to a gas of sodium and chlorine atoms.There are in fact two errors in such a simple approach. First, the argument has ignored the favourable energy of interaction between the cation and the anion. The net energy of formation of a Na+ion and a Cl−ion is the sum of three terms. The first is the energy investment needed to ionize a sodium atom. The second is a somewhat smaller energy that is released when the electron from the sodium atom attaches to a chlorine atom. At this stage, the net energy change is positive, indicating a higher energy than for the two atoms. However, because there is an attraction between opposite charges, there is a further release of energy as a result of the interaction of the two ions. This additional favourable contribution to the energy varies with the separation of the ions and strengthens as the two ions approach one another. Thus, at large separations the neutral atoms have the lowest energy, but as the two atoms are brought together a point is reached at which the lowest total energy is obtained if an electron transfers from the sodium atom to the chlorine atom. At this distance, and at shorter distances, Na+Cl−is the lower-energy species.The second feature omitted from the argument is that anionic compounddoes not consist of an isolatedcationandanion. An ionic compound is typically a solid formed from an array of alternating cations and anions. The packing of ions together and their electrostatic interactions with one another account for the typical features of ionic compounds—namely, their brittleness and high melting points. Moreover, when studying the stability of such compounds, one should more appropriately consider the energy changes associated with their formation from the elements in their standard state (such as solid metallic sodium and gaseous chlorine molecules) than from a gas of atoms of the elements.The Born-Haber cycleThe analysis of the formation of an ionic compound from its elements is commonly discussed in terms of a Born-Haber cycle, which breaks the overall process into a series of steps of known energy. The Born-Haber cycle for the formation ofsodium chloride is shown in Figure 5. At the start of the cycle, the elements are considered to be in the form in which they exist at normal pressure and temperature. First, sodium metal is vaporized to a gas of sodium atoms. This step requires an input of energy known as the atomization energy of sodium metal. Next, the appropriate number of chlorine molecules (Cl2) are broken apart to provide a gas of chlorine atoms. This step also requires a considerable input of energy that is called the dissociation energy of chlorine. The origin of these two contributions to the energy can be clarified by considering metallic and covalent bonding in more detail (specifically, the lowering of energy that occurs when metallic or covalent bonds form); here they can be treated as empirical quantities. At this stage, an electron is removed from each sodium atom and attached to each chlorine atom. The ionization requires a considerable input of energy, and a fraction of that investment is recovered from the electron affinity of the chlorine atoms. Overall, however, there is a considerable increase in energy as compared to the two starting materials.Born-Haber cycleFigure 5: The Born-Haber cycle for the formation of solid sodium chloride from solid sodium and chlorine gas. The energies involved (strictly, the enthalpies) are expressed in kilojoules per mole.Encyclopædia Britannica, Inc.At this stage, the ions are allowed to come together to form acrystallinearray. This step releases a large quantity of energy called thelattice energy of the compound. Energy is released in the process of crystalformation because first a cation becomes surrounded by anions, then that cluster of anions becomes surrounded by cations, and so on. As a result of this packing, every cation has anions as neighbours, and every anion has cations around it, and there is a strong overall attractive interaction among the many ions of opposite charge in the crystal. For sodium chloride, the lattice energy is so great that more energy is released in this step than is required for all the preceding steps combined, and solid sodium chloride therefore has a lower energy than sodium metal and chlorine gas. It is for this reason that, when sodium reacts with chlorine, a large quantity of heat is released.Factors favouring ionic bondingA Born-Haber cycle gives an indication of the factors that favour ionic bonding. Overall, the lattice energy must be great enough to overcome the energy required for ion formation. It follows that only elements with reasonably low ionization energies can contribute, as cations, to ionic materials, for too large an ionization energy could not be recovered from the resulting lattice energy. In practice, this criterion means that onlymetallicelements are likely to form cations, and two elements are unlikely to form an ionic compound unless one of them is a metal. Moreover, the steep increase in ionization energy required to break into a closed shell precludes the loss of all but the valence electrons. Furthermore, no more than about three electrons per atom can be lost before the increase in ionization energy becomes prohibitive.It can also be seen from the Born-Haber cycle that elements will contribute anions to an ionic compound only if their electron affinity is positive or, at least, not too strongly negative. Elements with positive electron affinities are likely to form anions (as long as a metal is present). A negative electron affinity can be tolerated provided it is not too great and the additional energy investment can be recovered from a greater lattice energy. That is the reason why ionicoxides are so common: although energy is required to push the second electron on an oxygen atom to make an O2−ion, the resulting ion produces such a high lattice energy that the energy investment is overcome.Ionic bonding is likely to occur if the lattice energy of the compound is large, for a large lattice energy can compensate for some strongly demanding energy requirements, most notably for cation formation, earlier in the cycle. High lattice energies are achieved if the ions that form the lattice are small and highly charged, for small ions can pack together closely and interact strongly with one another. The O2−ion of oxides is small (oxygen lies well to the right in the periodic table) and is reasonably highly charged (it has two negative charges; three negative charges is about the limit for monatomic anions). As a result, ionic oxides are widely formed by metallic elements. Although it is conceivable that O3−ions could be formed if enough energy were provided to overcome the repulsion from the many electrons present in O2−, the necessary energy would not be recovered from the lattice energy, for O3−anions are so large that the lattice energy of any compound they would form would be small. Once again, the termination of electron gain at a noble gas configuration is not so much a sign of some magic stability of such a species but rather a consequence of the fact that after such a configuration has been attained there is insufficient opportunity for achieving a lower energy.The actual pattern in which cations and anions pack together is the one that results in the greatest lattice energy (that is, the greatest lowering of energy of the ions relative to the gas of ions). For further details on crystal arrangements, see crystal.Covalent bondsWhen none of the elements in a compound is a metal, no atoms in the compound have an ionization energy low enough for electron loss to be likely. In such a case, covalence prevails. As a general rule, covalent bonds are formed between elements lying toward the right in the periodic table (i.e., the nonmetals). Molecules of identical atoms, such as H2and buckminsterfullerene (C60), are also held together by covalent bonds.Lewis formulation of a covalent bondIn Lewis terms a covalent bond is a sharedelectron pair. The bond between a hydrogen atom and a chlorine atom inhydrogen chloride is formulated as follows:In a Lewis structure of acovalent compound, the shared electron pair between the hydrogen and chlorine ions is represented by a line. The electron pair is called abonding pair;the three other pairs of electrons on the chlorine atom are calledlone pairs and play no direct role in holding the two atoms together.Each atom in the hydrogen chloride molecule attains a closed-shell octet of electrons by sharing and hence achieves a maximum lowering of energy. In general, an incomplete shell means that some attracting power of a nucleus may be wasted, and adding electrons beyond a closed shell would entail the energetic disadvantage of beginning the next shell of the atom concerned. Lewis’soctet rule is again applicable and is seen to represent the extreme means of achieving lower energy rather than being a goal in itself.A covalent bond forms if the bonded atoms have a lower total energy than the widely separated atoms. The simplest interpretation of the decrease in energy that occurs when electrons are shared is that both electrons lie between two attracting centres (the nuclei of the two atoms linked by the bond) and hence lie lower in energy than when they experience the attraction of a single centre. This explanation, however, requires considerable modification to capture the full truth about bonding, and it will be discussed further below when bonding is considered in terms of quantum mechanics.Lewis structures of more complex molecules can be constructed quite simply by extending the process that has been described for hydrogen chloride. First, the valence electrons that are available for bonding are counted (2 × 1 + 6 = 8 inH2O, for example, and 4 + 4 × 7 = 32 incarbon tetrachloride, CCl4), and the chemical symbols for the elements are placed in the arrangement that reflects which are neighbours:Next, one bonding pair is added between each linked pair of atoms:The remaining electrons are then added to the atoms in such a way that each atom has a share in an octet of electrons (this is the octet-rule part of the procedure):Finally, each bonding pair is represented by a dash:(Note that Lewis structures do not necessarily show the actual shape of the molecule, only the topological pattern of their bonds.)In some older formulations of Lewis structures, a distinction was made between bonds formed by electrons that have been supplied by both atoms (as in H−Cl, where one shared electron can be regarded as supplied by the hydrogen atom and the other by the chlorine atom) and covalent bonds formed when both electrons can be regarded as supplied by one atom, as in the formation of OH−from O2−and H+. Such a bond was called acoordinate covalent bond or a dative bond and symbolized O → H−. However, the difficulties encountered in the attempt to keep track of the origin of bonding electrons and the suggestion that a coordinate covalent bond differs somehow from a covalent bond (it does not) have led to this usage falling into disfavour.Advanced aspects of Lewis structuresThe Lewis structures illustrated so far have been selected for their simplicity. A number of elaborations are given below.Multiple bondsFirst, an atom may complete its octet by sharing more than one pair of electrons with a bonded neighbour. Two shared pairs of electrons, represented by a double dash (=), form adouble bond. Double bonds are found in numerous compounds, includingcarbon dioxide:Three shared pairs of electrons are represented by a triple dash (≡) and form atriple bond. Triple bonds are found in, for example,carbon monoxide,nitrogen molecules, andacetylene, shown respectively as:A double bond is stronger than a single bond, and a triple bond is stronger than a double bond. However, a double bond is not necessarily twice as strong as a single bond, nor is a triple bond necessarily three times as strong. Quadruple bonds, which contain four shared pairs of electrons, are rare but have been identified in some compounds in which two metal atoms are bonded directly together.ResonanceThere is sometimes an ambiguity in the location of double bonds. This ambiguity is illustrated by the Lewis structure forozone (O3). The following are two possible structures:In such cases, the actual Lewis structure is regarded as a blend of these contributions and is written:The blending together of these structures is actually a quantummechanical phenomenon called resonance, which will be considered in more detail below. At this stage, resonance can be regarded as a blending process that spreads double-bond character evenly over the atoms that participate in it. In ozone, for instance, each oxygen-oxygen bond is rendered equivalent by resonance, and each one has a mixture of single-bond and double-bond character (as indicated by its length and strength).HypervalenceLewis structures and theoctet rulejointly offer a succinct indication of the type of bonding that occurs in molecules and show the pattern of single and multiple bonds between the atoms. There are many compounds, however, that do not conform to the octet rule. The most common exceptions to the octet rule are the so-called hypervalent compounds. These are species in which there are more atoms attached to acentral atom than can be accommodated by an octet of electrons. An example issulfur hexafluoride, SF6, for which writing a Lewis structure with six S−F bonds requires that at least 12 electrons be present around the sulfur atom:(Only the bonding electrons are shown here.) In Lewis terms, hypervalence requires theexpansion of the octet to 10, 12, and even in some cases 16 electrons. Hypervalent compounds are very common and in general are no less stable than compounds that conform to the octet rule.The existence of hypervalent compounds would appear to deal a severe blow to the validity of the octet rule and Lewis’s approach to covalent bonding if the expansion of the octet could not be rationalized or its occurrence predicted. Fortunately, it can be rationalized, and the occurrence of hypervalence can be anticipated. In simple terms, experience has shown that hypervalence is rare in periods 1 and 2 of the periodic table (through neon) but is common in and after period 3. Thus, the octet rule can be used with confidence for carbon, nitrogen, oxygen, and fluorine, but hypervalence must be anticipated thereafter. The conventional explanation of this distinction takes note of the fact that, in period-3 elements, the valence shell has n = 3, and this is the first shell in whichdorbitals are available. (As noted above, these orbitals are occupied after the 4s orbitals have been filled and account for the occurrence of the transition metals in period 4.) It is therefore argued that atoms of this and subsequent periods can utilize the empty d orbitals to accommodate electrons beyond an octet and hence permit the formation of hypervalent species.In chemistry, however, it is important not to allow mere correlations to masquerade as explanations. Although it is true that d orbitals are energetically accessible in elements that display hypervalence, it does not follow that they are responsible for it. Indeed, quantum mechanical theories of the chemical bond do not need to invoke d-orbital involvement. These theories suggest that hypervalence is probably no more than a consequence of the greater radii of the atoms of period-3 elements compared with those of period 2, with the result that a central atom can pack more atoms around itself. Thus, hypervalence is more a steric (geometric) problem than an outcome of d-orbital availability. How six atoms can be bonded to a central atom by fewer than six pairs of electrons is discussed below.Incomplete-octet compoundsLess common than hypervalent compounds, but by no means rare, are species in which an atom does not achieve an octet of electrons. Such compounds are called incomplete-octet compounds. An example is the compoundboron trifluoride, BF3, which is used as an industrial catalyst. The boron (B) atom supplies three valence electrons, and a representation of the compound’s structure is:The boron atom has a share in only six valence electrons. It is possible to write Lewis structures that do satisfy the octet rule.However, whereas in the incomplete octet structure the fluorine atoms have three lone pairs, in these resonance structures one fluorine atom has only two lone pairs, so it has partly surrendered an electron to the boron atom. This is energetically disadvantageous for such an electronegative element as fluorine (which is in fact the most electronegative element), and the three octet structures turn out to have a higher energy than the incomplete-octet structure. The latter is therefore a better representation of the actual structure of the molecule. Indeed, it is exactly because the BF3molecule has an incomplete-octet structure that it is so widely employed as a catalyst, for it can use the vacancies in the valence shell of the boron atom to form bonds to other atoms and thereby facilitate certain chemical reactions.Electron-deficient compoundsAnother type of exception to the Lewis approach to bonding is the existence of compounds that possess too few electrons for a Lewis structure to be written. Such compounds are called electron-deficient compounds. A prime example of an electron-deficient compound isdiborane, B2H6. This compound requires at least seven bonds to link its eight atoms together, but it has only 2 × 3 + 6 × 1 = 12 valence electrons, which is enough to form only six covalent bonds. Once again, it appears that, as in hypervalent compounds, the existence of electron-deficient compounds signifies that a pair of electrons can bond together more than two atoms. The discussion of the quantum mechanical theory of bonding below shows that this is indeed the case.A number of exceptions to Lewis’s theory of bonding have been catalogued here. It has further deficiencies. For example, the theory is not quantitative and gives no clue to how the strengths of bonds or their lengths can be assessed. In the form in which it has been presented, it also fails to suggest the shapes of molecules. Furthermore, the theory offers no justification for regarding anelectron pair as the central feature of a covalent bond. Indeed, there are species that possess bonds that rely on the presence of a single electron. (The one-electron transientspecies H2+is an example.) Nevertheless, in spite of these difficulties, Lewis’s approach to bonding has proved exceptionally useful. It predicts when the octet rule is likely to be valid and when hypervalence can be anticipated, and the occurrence of multiple bonds and the presence of lone pairs of electrons correlate with the chemical properties of a wide variety of species. Lewis’s approach is still widely used as a rule of thumb for assessing the structures and properties of covalent species, and modern quantum mechanical theories echo its general content.The following sections discuss how the limitations of Lewis’s approach can be overcome, first by extending the theory to account for molecular shapes and then by developing more thorough quantum mechanical theories of the chemical bond.Molecular shapes andVSEPR theoryThere is a sharp distinction betweenionic andcovalent bonds when the geometric arrangements of atoms in compounds are considered. In essence, ionic bonding is nondirectional, whereas covalent bonding is directional. That is, in ionic compounds there is no intrinsically preferreddirection in which a neighbour should lie for the strength of bonding to be maximized. In contrast, in a covalently bonded compound, the atoms adopt specific locations relative to one another, as in thetetrahedral arrangement of hydrogen atoms around the central carbon atom in methane, CH4, or theangular arrangement of atoms in H2O.The lack of directionality of ionic bonds stems from the isotropy (spherical symmetry) of the electrostatic forces between ions. As has already been pointed out, the result of this isotropy is that ionsstack together in the locations necessary to achieve the lowest energy and in this way give rise to the common packing patterns characteristic of many ionic solids. When deviations from stacking schemes are observed that seem to indicate that the ions are being held in certain orientations relative to their neighbours, it is a sign that covalent bonding is beginning to influence the structure of the solid and that the bonding is not purely ionic. This is the case, for example, in the compoundnickel arsenide (NiAs), which has a structure that suggests that a degree of covalent bonding is present (Figure 6). It is fully apparent in the structure of diamond (Figure 7), in which each carbon atom is in a tetrahedral position relative to its neighbour and in which the bonding is essentially purely covalent.nickel arsenideFigure 6: The crystal structure of nickel arsenide. This type of structure departs strongly from that expected for ionic bonding and shows the importance of covalence. There is also some direct nickel-nickel bonding that tends to draw the nickel atoms together.Encyclopædia Britannica, Inc.diamondFigure 7: The crystal structure of diamond. Each carbon atom is bonded covalently to four neighbours arranged tetrahedrally around the central atom. The structure is highly rigid.Encyclopædia Britannica, Inc.The rationalization of the structures adopted by purely ionic solids is essentially a straightforward exercise in the analysis of electrostatic interactions between ions. The problem of the structures of covalent compounds, both individual molecules, such as methane, and covalently bonded solids, such as diamond, is much more subtle, for it involves delving into the characteristics of the electron arrangements in individual atoms. Thus, if the formation of a covalent bond is regarded as corresponding to the accumulation of electrons in a particular region of an atom, then, to form a second bond, electrons can be accumulated into only certain parts of the atom relative to that first region of enhanced electron density. As a result, the bonds will lie in a geometric array that is characteristic of the atom. The remainder of this section focuses on this problem, but a detailed quantum mechanical analysis is required for a full understanding of the matter.The theory of molecular shape known as valence-shell electron-pair repulsion (VSEPR) theory grew out of Lewis’s theory, and, like that approach to bonding, VSEPR focuses on the role of electron pairs. It stems from the work of the British chemists H.M. Powell and Nevil V. Sidgwick in the 1940s and was extensively developed by R.J. Gillespie in Canada and Ronald S. Nyholm in London during the 1960s. As such, it postdates quantum mechanical theories of bonding and shape but should be seen (as is so common a motivation in chemistry) as an attempt to identify the essential features of a problem and to formulate them into a simple qualitative procedure for rationalization and prediction.A Lewis structure, as shown above, is a topological portrayal of bonding in a molecule. It ascribes bonding influences toelectron pairs that lie between atoms and acknowledges the existence of lone pairs of electrons that do not participate directly in the bonding. The VSEPR theory supposes that all electron pairs, both bonding pairs and lone pairs, repel each other—particularly if they are close—and that the molecular shape is such as to minimize these repulsions. The approach is commonly applied to species in which there is an identifiable central atom (the oxygen atom in H2O, for instance), but it is straightforward to extend it to discussions of the local shape at any given atom in a polyatomic species.Applying VSEPR theory to simple moleculesThemethane molecule, CH4, can be used to illustrate the procedure for predicting molecular shape. The Lewis structure of this molecule ascribes four bonding electron pairs to the carbon atom (Figure 8). These pairs repel one another, and their separation is maximized if they adopt a tetrahedral dispositionaround the central carbon atom. A hydrogen atom is attached by each bonding pair, so it can be predicted that CH4is likely to be a tetrahedral species, which is in fact the case.methaneFigure 8: The structure of methane, CH4. This regular tetrahedral structure is explained in the VSEPR theory of molecular shape by supposing that the four pairs of bonding electrons (represented by the gray clouds) adopt positions that minimize their mutual repulsion.Encyclopædia Britannica, Inc.When applying VSEPR theory, attention is first focused on the electron pairs of thecentral atom, disregarding the distinction between bonding pairs and lone pairs. These pairs are then allowed to move around the central atom (at a constant distance) and to take up positions that maximize their mutual separations. As in the methane molecule, four pairs adopt a tetrahedral disposition. The arrangements adopted by two through six pairs are summarized in the table. At this stage, the atoms that are attached by the bonding pairs are introduced, and the shape of the molecule is reported on the basis of the arrangement of these atoms.Thewater molecule, H2O, provides a simple example. The oxygen atom has four electron pairs, so these pairs adopt a tetrahedral arrangement. Two of the pairs are bonding, and hydrogen atoms are attached to them. Hence, the molecule is angular. (Note that the shape of the molecule is determined by the disposition of the atoms, not the disposition of the electron pairs.) Theammonia molecule, NH3, has four electron pairs in a tetrahedral arrangement around the nitrogen atom; three of these pairs are used to bond hydrogen atoms, so the molecule is predicted to betrigonal pyramidal, with a lone pair in the apical position. Some of the names of the shapes of simple molecules are summarized in the table.electron pairs and molecular shapeVSEPR electron pair arrangements and associated molecular shapes.Encyclopædia Britannica, Inc.Theangle between electron pairs in a tetrahedral arrangement is 109.5°. However, although H2O is indeed angular and NH3is trigonal pyramidal, the angles between the bonds are 104° and 107°, respectively. In a sense, such close agreement is quite satisfactory for so simple an approach, but clearly there is more to explain. To account for variations in bond angle, it is supposed that electron pair repulsions are greatest between lone pairs, less between lone pairs and bonding pairs, and least between bonding pairs. The justification of this ordering has proved somewhat elusive; qualitatively it is presumed that lone pairs, being attached only to a single centre, spread over a greater volume than bonding pairs, which are pinned between two attracting centres. Whatever the reason may be, the order correlates quite well with observation. Thus, in H2O the two lone pairs move apart a little, and the two bonding pairs move away from them by closing the angle between one another. Likewise, in NH3the three bonding pairs move back from the single lone pair to minimize their interaction with it. As a result, the H−N−H bond angle decreases slightly. In each case, the predicted angle is less than the tetrahedral angle, as is observed experimentally.VSEPR theory is quite successful at predicting (or at least rationalizing) the overall shapes of molecules. Thus, the hypervalent species SF6(sulfur hexafluoride), with six bonding pairs, is predicted and found to be a regular octahedron, and PCl5(phosphoruspentachloride), with five bonding pairs, is predicted and found to be atrigonal bipyramid. The XeF4(xenon tetrafluoride) molecule is hypervalent with six electron pairs around the central xenon (Xe) atom. These pairs adopt anoctahedral arrangement. Four of the pairs are bonding pairs, and two are lone pairs. According to VSEPR theory, the repulsion between the lone pairs is minimized if they lie on opposite sides of the xenon atom, leaving the four equatorial pairs as bonding pairs.This analysis suggests that XeF4should be a planar species, which is found to be the case.Molecules withmultiple bondsThere are further rules in VSEPR theory that simplify the discussion of species with multiple bonds and of species in which resonance must be considered. An analysis of the shapes adopted by species with multiple bonds suggests that each multiple bond can be treated as a single “superpair” of electrons. This rule can be justified by considering the geometric shapes that stem from two atoms sharing two or more pairs of electrons (Figure 9). Thus, thesulfate ion, SO42−, for which a Lewis structure ischemical bondFigure 9: Double bonds. The geometric arrangement of atoms linked by two shared pairs of electrons in a double bond (top) can be simulated by treating the double bond as the result of the sharing of a single superpair of electrons (bottom).Encyclopædia Britannica, Inc.can be treated as having the equivalent of four pairs (two ordinary pairs and two superpairs) around the sulfur atom in a tetrahedral arrangement. All four pairs arebonding, so the ion is predicted to be a regular tetrahedron, which it indeed is. The same conclusion about the shape of the molecule would be drawn from another possible Lewis structure, in which each bond is single:The actual molecule is aresonance hybrid of these and related structures; but, as each one corresponds to the same geometry, no particular Lewis structure need be selected before one can make a prediction based on VSEPR theory. In other words,resonance does not affect the shapes of molecules.Molecules with nocentral atomExamples of the manner in which VSEPR theory is applied to species in which there is no central atom are provided byethane (C2H6),ethylene(C2H4), andacetylene (C2H2), the Lewis structures for which are, respectively, the following:In each case, consider the local environment of each carbon atom. In ethane there are four bonding pairs around each carbon atom, so every carbon atom is linked to its four neighbours (one carbon atom and three hydrogen atoms) by a tetrahedral array of bonds. The bond angles in ethane are indeed all close to 109°. In ethylene each carbon atom possesses two ordinary bonding pairs (linking it to hydrogen atoms) and one superpair (linking it to the other carbon atom). These three pairs, and the corresponding bonds, adopt aplanar triangular arrangement, and the H−C−H and H−C=C angles are predicted to be close to 120°, as is found experimentally. It is less apparent from this analysis, but understandable once it is realized that the superpair is actually two shared pairs (Figure 9), that the ethylene molecule is predicted to be planar. Each carbon atom in an acetylene molecule has one bonding pair (to hydrogen) and one superpair (to the other carbon atom). The molecule is therefore expected to belinear, as is found in practice. The linearity of the molecule can be appreciated by referring to Figure 9.Limitations of the VSEPR modelThe VSEPR theory is simple yet powerful. Nevertheless, like any simplified model, it has its limitations. First, although it predicts that the bond angle in H2O is less than the tetrahedral angle, it does not make any attempt to predict the magnitude of the decrease. Second, the theory makes no predictions about the lengths of the bonds, which is another aspect of the shape of a molecule. Third, it ascribes the entire criterion of shape to electrostatic repulsions between bonding pairs, when in fact there are numerous contributions to the total energy of a molecule, and electrostatic effects are not necessarily the dominant ones. Fourth, the theory relies on some vague concepts, such as the difference in repelling effects of lone pairs and bonding pairs. There also are some species for which VSEPR theory fails. Nevertheless, despite these limitations and uncertainties, VSEPR theory is a useful rule of thumb and can be used with reasonable confidence for numerous species.Thepolarity of moleculesThere are three main properties of chemical bonds that must be considered—namely, their strength, length, and polarity. The polarity of a bond is the distribution of electrical charge over the atoms joined by the bond. Specifically, it is found that, while bonds between identical atoms (as in H2) are electrically uniform in the sense that both hydrogen atoms are electrically neutral, bonds between atoms of different elements are electrically inequivalent. Inhydrogen chloride, for example, the hydrogen atom is slightly positively charged whereas the chlorine atom is slightly negatively charged. The slight electrical charges on dissimilar atoms are called partial charges, and the presence of partial charges signifies the occurrence of a polar bond.The polarity of a bond arises from the relative electronegativities of the elements.Electronegativity, it will be recalled, is the power of an atom of an element to attract electronstoward itself when it is part of a compound. Thus, although a bond in a compound may consist of a shared pair of electrons, the atom of the more electronegative element will draw the shared pair toward itself and thereby acquire a partial negative charge. The atom that has lost its equal share in the bonding electron pair acquires a partial positive charge because its nuclear charge is no longer fully canceled by its electrons.The existence of equal but opposite partial charges on the atoms at each end of a heteronuclear bond (i.e., a bond between atoms of different elements) gives rise to anelectric dipole. The magnitude of this dipole is expressed by the value of itsdipolemoment, μ, which is defined as the product of the magnitude of the partial charges times their separation (essentially, the length of the bond). The dipole moment of a heteronuclear bond can be estimated from the electronegativities of the atoms A and B, χAand χB, respectively, by using the simple relationwhere D denotes the unit debye, which is used for reporting molecular dipole moments (1 D = 3.34 × 10−30coulomb·metre). Moreover, the negative end of the dipole lies on the more electronegative atom. If the two bonded atoms are identical, it follows that the dipole moment is zero and the bond is nonpolar.As the difference in electronegativity between two covalently bonded atoms increases, the dipolar character of the bond increases as the partial charges increase. When the electronegativities of the atoms are very different, the attraction of the more electronegative atom for the shared electron pair is so great that it effectively exercises complete control over them. That is, it has gained possession of the pair, and the bond is best regarded asionic. Ionic andcovalent bonding therefore can be regarded as constituting a continuum rather than as alternatives. This continuum can be expressed in terms of resonance by regarding a bond between atoms A and B as a resonance between a purely covalent form, in which the electrons are shared equally, and a purely ionic form, in which the more electronegative atom (B) has total control over the electrons:As the electronegativity difference increases, the resonance lies increasingly in favour of the ionic contribution. When the electronegativity difference is very large, as between an electropositive atom like sodium and an electronegative atom like fluorine, the ionic structure dominates the resonance, and the bonding can be regarded as ionic. Thus, as the electronegativity difference of the two bonded elements increases, a nonpolar bond gives way to a polar bond, which in turn becomes an ionic bond. There are, in fact, no purely ionic bonds, just as there are no purely covalent bonds; bonding is a continuum of types.Even a homonuclear bond, which is a bond between atoms of the same element (as inCl2), is not purely covalent, because a more accurate description would be in terms ofionic-covalent resonance:That the species is nonpolar despite the occurrence of ionic contributions stems from the equal contributions of the ionic structures Cl−Cl+and Cl+Cl−and their canceling dipoles. That Cl2is commonly regarded as a covalently bonded species stems from the dominant contribution of the structure Cl−Cl to this resonance mixture. In contrast, the valence bond theory wave function (see below Valence bond theory) of hydrogen chloride would be expressed as the resonance hybridIn this case, the two ionic structures would contribute different amounts (because the elements have different electronegativities), and the larger contribution of H+Cl−is responsible for the presence of partial charges on the atoms and the polarity of the molecule.Apolyatomic molecule will have polar bonds if its atoms are not identical. However, whether or not the molecule as a whole is polar (i.e., has a nonzero electric dipole moment) depends on the shape of the molecule. For example, the carbon-oxygen bonds incarbon dioxide are both polar, with the partial positive charge on the carbon atom and the partial negative charge on the more electronegative oxygen atom. The molecule as a whole is nonpolar, however, because the dipole moment of one carbon-oxygen bond cancels the dipole moment of the other, for the two bond dipole moments point in opposite directions in this linear molecule. In contrast, thewatermolecule is polar. Each oxygen-hydrogen bond is polar, with the oxygen atom bearing the partial negative charge and the hydrogen atom the partial positive charge. Because the molecule is angular rather than linear, the bond dipole moments do not cancel, and the molecule has a nonzero dipole moment.The polarity of H2O is of profound importance for the properties of water. It is partly responsible for the existence of water as a liquid at room temperature and for the ability of water to act as a solvent for many ionic compounds. The latter ability stems from the fact that the partial negative charge on the oxygen atom can emulate the negative charge of anions that surround each cation in the solid and thus help minimize the energy difference when the crystaldissolves. The partial positive charge on the hydrogen atoms can likewise emulate that of the cations surrounding the anions in the solid.The quantum mechanics of bondingIntermolecular forcesVarieties of solidsAdvanced aspects of chemical bondingMore About This TopicView All Media (28)Additional ReadingExternal WebsitesArticle HistoryArticle ContributorsKeep ExploringRelated Britannica ArticlesChemical associationChemical compoundChemistryElectronegativityHydrogen bondingIrving LangmuirMoleculeLinus PaulingTheory of resonanceVan der Waals forces
- Home >
- Catalog >
- Education >
- Chemistry Chart >
- Electron Configuration Chart >
- Electron Configurations Of Atoms In The Ground State >
- Arrangement Of Electrons In Atoms Section 1 The Development