How to Edit The Be Able To Describe Periodic Trends Why Do Ions freely Online
Start on editing, signing and sharing your Be Able To Describe Periodic Trends Why Do Ions online refering to these easy steps:
- click the Get Form or Get Form Now button on the current page to make access to the PDF editor.
- hold on a second before the Be Able To Describe Periodic Trends Why Do Ions is loaded
- Use the tools in the top toolbar to edit the file, and the edited content will be saved automatically
- Download your modified file.
A top-rated Tool to Edit and Sign the Be Able To Describe Periodic Trends Why Do Ions
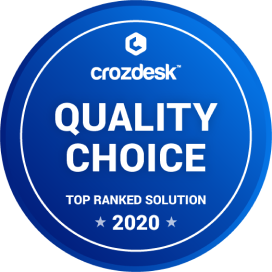
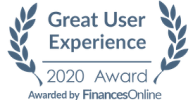
A clear guide on editing Be Able To Describe Periodic Trends Why Do Ions Online
It has become very simple these days to edit your PDF files online, and CocoDoc is the best free app you have ever used to make some editing to your file and save it. Follow our simple tutorial to try it!
- Click the Get Form or Get Form Now button on the current page to start modifying your PDF
- Add, modify or erase your content using the editing tools on the toolbar on the top.
- Affter editing your content, put the date on and make a signature to finish it.
- Go over it agian your form before you click to download it
How to add a signature on your Be Able To Describe Periodic Trends Why Do Ions
Though most people are in the habit of signing paper documents by writing, electronic signatures are becoming more normal, follow these steps to sign documents online for free!
- Click the Get Form or Get Form Now button to begin editing on Be Able To Describe Periodic Trends Why Do Ions in CocoDoc PDF editor.
- Click on the Sign icon in the tool menu on the top
- A box will pop up, click Add new signature button and you'll have three options—Type, Draw, and Upload. Once you're done, click the Save button.
- Move and settle the signature inside your PDF file
How to add a textbox on your Be Able To Describe Periodic Trends Why Do Ions
If you have the need to add a text box on your PDF in order to customize your special content, take a few easy steps to carry it throuth.
- Open the PDF file in CocoDoc PDF editor.
- Click Text Box on the top toolbar and move your mouse to carry it wherever you want to put it.
- Fill in the content you need to insert. After you’ve writed down the text, you can use the text editing tools to resize, color or bold the text.
- When you're done, click OK to save it. If you’re not settle for the text, click on the trash can icon to delete it and start again.
An easy guide to Edit Your Be Able To Describe Periodic Trends Why Do Ions on G Suite
If you are seeking a solution for PDF editing on G suite, CocoDoc PDF editor is a suggested tool that can be used directly from Google Drive to create or edit files.
- Find CocoDoc PDF editor and set up the add-on for google drive.
- Right-click on a chosen file in your Google Drive and choose Open With.
- Select CocoDoc PDF on the popup list to open your file with and give CocoDoc access to your google account.
- Make changes to PDF files, adding text, images, editing existing text, annotate in highlight, fullly polish the texts in CocoDoc PDF editor before saving and downloading it.
PDF Editor FAQ
Where/how can I learn core chemistry concepts?
Well, chemistry has a few core concepts:Chemical Nomenclature: how to name atoms, molecules, or compounds correctly.Atomic Structure: structure plays big role in chemistry.Periodic Trends: this is also very important. not memorize the table, but understand things like electronegativity, ion affinity, atomic size, etc.,Chemical Reactions: understand the basic reactions, there are not that many at introductory level, but definitely be able to recognize combustion reaction.Stoichiometry: be able to balance a chemical equation and how to look at an equation to perform dimensional analysis. That means, to go from grams products to grams of reactants and vice versa. To convert from grams to moles and vice versa. This is very very very useful.Acid-Base Chemistry: this is very important as well. As almost all reactions are acid-base. Understand the various types of bases, pH, water pH, what describes an acid and a base.I think it’s useful to understand these 6 core concepts and learn them in order from 1 to 6.You shouldn’t have to spend 5 hours studying chemistry at the general level. It sort of sounds like you made the same mistake I did when I was 15, which was focus on memorizing as much as I can. Unfortunately, you can’t do that in Chemistry because it is an applied science; it is not biology and it does require some thinking. Granted, there are rules that you must memorize, but, it’s more important to understand the rules so you can apply it.For example, memorizing the periodic table won’t help you explain why fluorine is smaller than oxygen. So, just think about this, and it will help.Good Luck! :)
Why is HF a weak acid?
TLDR:HF is a weak acid, all other hydrohalic acids are strong! Just accept it!Corrosiveness and acidity are two different subjects. HF is very corrosive because fluor -as the most electronegative element -can form stable compounds with almost everything.Regarding the acidity of HF, I’m afraid it is not so easy to explain. I’m not claiming that this answer will solve all questions. (But at least I can try.)The question is puzzling chemists for a very long time. In 1956 Linus Pauling himself already devoted an article on the subject (Why is hydrofluoric acid a weak acid? J. Chem. Educ., 1956, 33 (1), p 16)I will start with some general definitions.Brønsted-Lowry acidity is a matter of equilibrium (in this case with water.)HX(aq)+ H[math]_2[/math]O(l) ⇌ H[math]_3[/math]O[math][/math][math]^+[/math] (aq) + X[math]^-[/math](aq)This equilibrium is quantified via Kₐ, the acid dissociation constant. Chemists often use the negative logarithm of this constant.pK[math]_a[/math] = -log[math]_{10}[/math]K[math]_a[/math].The lower the pKₐ value, the stronger the acid.If we tabulate pKₐ of the hydrohalic acids:[math]\small{\begin{array}{cccc} \hline \text{HX} & \text{pK}_a \\\hline \text{HF} & 3.2 [/math][math][/math][math]\\ \text{HCl} & -5.9 ± 0.4 [/math][math][/math][math]\\ \text{HBr} & -8.8 ± 0.8 [/math][math][/math][math]\\ \text{HI} & -9.5 ± 1.0 [/math][math][/math][math]\\ \hline \end{array}}[/math]HF is the only weak acid all others are strong acids.The simplistic definition of a strong acid is ‘any acid that ionizes completely in solution’, but more formally it is an acid with a pKₐ value smaller than the hydronium ion. Or in other words, a strong acid has a negative pKₐ.Actually, a negative pKₐ means that the concentrations of HX in the above equilibrium are very small. Too small to measure, therefore the pKₐ value of HCl, HBr, and HI are not that reliable.I took the values from a 2016 article (J. Phys. Chem. A 2016, 120, pp 3663−3669) and it includes the potential errors. Please note that these values are logarithmic so the error of +/- 1 pKₐ units on HI actually implicates a factor of 10 on the Kₐ.Regardless of the values, it is clear that there is a large difference between HF and the other hydrohalic acids.If we compare this to another series: from methane CH₄ (or H-CH₃) to HF (i.e. moving left to right in the periodic table)[math]\small{\begin{array}{cccc} \hline \text{H-X} & \text{pK}_a & \chi \\\hline \text{H-CH}_3 & \sim 50 & 2.5\\ \text{H-NH}_2 & 35 & 3.0\\ \text{H-OH} & 14.0 & 3.5 [/math][math][/math][math]\\ \text{H-F} & 3.2 & 4.0\\ \hline \end{array}}[/math]The difference in acidity is nicely correlated to the difference in electronegativity of the different elements. A difference in electronegativity makes the bond more polar and this fits nicely with the trend in acidity: you indeed expected to have a greater tendency to go to the anionic form.But if we do the same when we down the table we should be able to make the same argument. Fluor is the most electronegative element and the bond is indeed more polar. But the difference in acidity is totally reversed.[math]\small{\begin{array}{cccc} \hline \text{HX} & \text{pK}_a & \chi \\\hline \text{HF} & 3.2 & 4.0 [/math][math][/math][math]\\ \text{HCl} & -5.9 & 3.0\\ \text{HBr} & -8.8 & 2.8 [/math][math][/math][math]\\ \text{HI} & -9.5 & 2.5 [/math][math][/math][math]\\ \hline \end{array}}[/math]This is a bit puzzling! Why would we use electronegativity to explain the difference in acidity in one case (moving horizontally) but completely disregard it if we go down the periodic table?Some explain it by the bond strength and claim that HF has the stronger bond in the series of HF to HI and therefore correlate acidity with bond strength.The reason for HF’s meager acidity is the strong bond between the hydrogen and the fluorine atom, resulting in only partial ionization in dilute solutions. (Why Hydrofluoric Acid Is a Weak Acid)The fact that the HF bond is stronger is correct.I tabulated them (data from Acc. Chem. Res., 2003, 36 (4), pp 255–263)[math]\small{\begin{array}{cccc} \hline \text{HX} & \text{pK}_a & \Delta H_{bond} \text{/kJ mol}^{-1} \\\hline \text{HF} & 3.2 & 571 [/math][math][/math][math]\\ \text{HCl} & -5.9 & 432\\ \text{HBr} & -8.8 & 367 [/math][math][/math][math]\\ \text{HI} & -9.5 & 299 [/math][math][/math][math]\\ \hline \end{array}}[/math]But the bond strenght explanation is again problematic and confusing. Especially if we would check the bond dissociation enthalpy in the series from CH₄ to HF. In that series the strongest bond gives the strongest acidl![math]\small{\begin{array}{cccc} \hline \text{HX} & \text{pK}_a & \Delta H_{bond} \text{/kJ mol}^{-1} \\\hline \text{H-CH}_3 & \sim 50 & 440 [/math][math][/math][math]\\ \text{H-NH}_2 & 35 & 451\\ \text{H-OH} & 14.0 & 498 [/math][math][/math][math]\\ \text{H-F} & 3.2 & 571 [/math][math][/math][math]\\ \hline \end{array}}[/math]Why would we use bond strength to explain the difference in acidity if we go down the periodic table but completely disregard if we move horizontally?It is almost like we can claim.[math]\boxed{\text{The main purpose of chemistry is [/math][math]to[/math][math] confuse students.}}[/math]In all seriousness. We are dealing with acidity in solution and therefore we can’t simplify this using one set of data.To start with the above data of the bond dissociation enthalpy is the energy required for homolytic bond cleavage into its two radicals. (We are interested in heterolytic bond cleavage or formations of ions!). In addition, this is thermodynamic data in the gas phase (not in water!).So it is clear that bond energy alone can not give you enough information.The full process can be described via a thought process using so-called Born–Haber cycles.Figure 1: Born-Haber Cycle of ion-formation of the halogen acids.Going from the neutral species in water we first need to remove it from water to bring it to the gas phase. Subsequently, cleave the bond (bond dissociation energy) and ionize (Ionization energy for hydrogen, Electron Affinities for X) As the last step, the ions are hydrated again.But we immediately come into serious problems using this thought process: we lack the data of all these steps. Actually, this is not that unexpected the data on the dehydration faces the same problem as a measurement of the concentration. The concentration of the neutral species is far too low in water, so thermodynamic data on the dehydration is not available. (For HF it is available, for the others, it might be not so reliable.)I tabulated the data coming from various sources. Electron affinity from Wikipedia, Bond energies from the above source, and dehydration energies from Ayotte’s article. (See below for the reference.)Table 1: Enthalpy changes for the Born Haber cycle of acidity of HX (see Figure 1 for codes)The total enthalpy difference includes values for Ionization Energy of hydrogen (1318.4 kJ/mol) and the hydration enthalpy of H+ (-1094 kJ/mol)As discussed previously the Bond energy (2) goes down towards Iodine. Also, the Electron Affinities (3) goes down towards Iodine, although F is not following the trend:The incoming electron is going to be closer to the nucleus in fluorine than in any other of these elements, so you would expect a high value of electron affinity. However, because fluorine is such a small atom, you are putting the new electron into a region of space already crowded with electrons and there is a significant amount of repulsion. This repulsion lessens the attraction the incoming electron feels and so lessens the electron affinity. A similar reversal of the expected trend happens between oxygen and sulfur in Group 16. The first electron affinity of oxygen (-142 kJ mol-1 ) is smaller than that of sulfur (-200 kJ mol-1) for exactly the same reason that fluorine’s is smaller than chlorine's.Why is Fluorine an Anomaly?Looking at the data one thing is striking: the difference in bond energy (2) is almost completely compensated by the difference the energy released by hydration (4) of the halogen ions.The total enthalpy change (ΔH total) seems to be negative for all halogens. Meaning it is an exothermic reaction for all of them, although overall the process is less exothermic for fluor.This lower exothermicity for the ionization of fluor in water is — based on the data -- mainly due to the differences in the first step: it cost more energy to dehydrate HF (1).Similar to ammonia and water, hydrogen fluoride is capable of forming strong hydrogen-bonds (with itself, but also with water). It costs a lot of energy to overcome these hydrogen bonds. This is e.g. also the reason for the higher boiling points of water, ammonia, and hydrogen fluoride.(scheme from Chemguide - intermolecular bonding - hydrogen bonds)Anyhow the process is exothermic for all of the hydrohalic acids. In order to explain the difference in acidity we need to dig further into the data:Based on the pKa values as quoted previously. We can calculate the total difference of Gibbs free energy. Using the formula ΔG° = 2.303 RT pKₐAnd the total entropy change. (based on ΔG° = ΔH° - TΔS°)Table 2: Gibbs Free energy and entropy changesBased on these values we can conclude that the true reason for the fact that HF is a weak base is mainly due to entropic reasons.The total ΔS° in the above table is the entropy for the full cycle but based on CRC handbook the standard entropy S° of aqueous F⁻ is –13.8 J/mol.K (compared to values of 56.5, 82.4, and 111.3 J/mol.K for Cl⁻, Br⁻ and I⁻)The fluor ion is small and has a large charge density. The solvation of fluor ions in water, therefore, produces a significant order in the surrounding water (and hydronium) molecules. Hence a negative entropy. This effect is much less in the larger halogen ions.This effect is demonstrated experimentally by Ayotte and coworkers in ‘Why is hydrofluoric acid a weak acid?’At lower temperature, the entropic part of the Gibbs free energy is indeed of lesser importance (ΔG° = ΔH° - TΔS°). By using infrared vibrational spectra at a very low temperature (40K) they could demonstrate that HF is indeed a much stronger acid at these lower temperatures.To be complete: There are alternatives explanations as well:There is good spectrocsopic evidence that hydrogen fluoride ionizes fairly completely in solution in water, but instead of producing free hydroxonium ions, H₃O+, and fluoride ions, there is such strong attraction between these that they form a strongly bound ion pair, H₃O+.F-Hydrogen halides as acidsAlthough other researchers have questioned that observation.I already tried to explain the difference between corrosivity and acidity in another answer so please read Kurt VdB's answer to What makes an acid so corrosive?
Why would you support, or not support, only using nuclear energy?
Yes I believe that nuclear power should become the world’s primary energy/power source and will give some reasons why. A few months ago I was asked to write/contribute a chapter for an upcoming soil science book describing how a "nuclear renaissance" might address Africa’s impending agricultural catastrophes. Since a properly implemented renaissance could address the entire world’s long term agricultural issues, I dreamed up a scenario akin to that envisioned by two of ORNL’s leading lights a long time ago emphasizing two “apps” that they hadn’t mentioned – simultaneous soil replenishment & atmospheric CO2 sequestration via the "enhanced weathering" of powdered basalt. This QUORA answer is excerpted from that effort.Warning – I’m a comfortably retired US National Laboratory scientist who spent most of his last decade at work “whistleblowing”– if you want soothing words and political correctness, read something else.IntroductionBecause I am among the many (Springer 2014, Sims 2011, Hansen 2008, Hansen 2016, and EFN 2018 ) who feel that today’s politically correct (non-nuclear) renewable energy sources couldn’t support even the near future’s (2050 AD) anticipated human population without severe environmental consequences, this essay invokes a future similar to that envisioned by Oak Ridge National Laboratory’s (ORNL’s) H. E. Goeller and Alvin Weinberg over forty years ago (Goeller and Weinberg 1976). In this scenario, by 2100 AD, a “sustainable nuclear renaissance” – not an “all of the above” mix of politically correct energy sources - would be addressing the root causes of mankind’s squabbling over resources responsible for most of humanity’s misery both now and in the past. Among other things, it would simultaneously reverse environmental degradation and render food production sustainable by enabling the mining, grinding, shipping, and distribution of powdered basalt over farmland thereby affecting Mother Nature’s too slow, notoriously unreliable and sometimes catastrophic (volcanic) approach to both soil-building and CO2 sequestration. Wherever possible I’ll support my arguments with simple calculations utilizing reasonable assumptions and readily obtained data, not sweeping generalizations or wishful thinking. I’ll also put the resulting numbers into proper perspective -“naked” numbers – especially very large or small ones - don’t mean much to most people including scientists & engineers. Since the book for which the original version of this essay was written is devoted to addressing Africa’s soil/agriculture issues, most of my examples will involve calculations relevant to that continent’s problems. Finally, since it currently seems unlikely that either Africa or its Western world “helpers” will develop/implement a nuclear renaissance capable of powering this scenario , I will also discusses the most likely way that it might actually be realized – China will take the lead.Africa’s Especially Special IssuesUnlike most first world nations most of Africa’s 54 countries continue to exhibit alarmingly high rates of both population growth and poverty (ESA 2015). Approximately 380 million of its ~1.2 billion people are extremely poor – often hungry – and ten of the world’s most underdeveloped (“shithole”, Trump 2018) countries – Mozambique, Guinea, Burundi, Burkina Faso, Eritrea, Sierra Leone, Chad, Central African Republic, Democratic Republic of Congo, and Niger – are located therein. Although considered exceptionally underdeveloped, none of them are among the twenty with the world’s lowest living costs (Cheap 2017) meaning that Africa’s poor people are considerably poorer than are those in more technologically advanced but poor by OECD standards nations like Romania. Africa’s people are plagued by a lack of basic infrastructure due to dysfunctional and, often, self-serving governance further complicated by long festering civil/tribal/religious conflicts and therefore most of them face bleak futures. The fact that such countries are ill equipped to deal with any sort of natural disaster, possess economies comprised primarily of subsistence farming on progressively poorer-quality land, and have grossly underfunded public health, physical infrastructure, and education services constitute only some of the factors considered in compiling quality-of-life rankings. Most of the United Nation’s measures of Human Development (UNDP 2018) also consider the fairness of income/wealth distribution for which factor Africa’s countries are also especially low-ranking (GINI 2018). Cambridge’s Sir Partha Dasgupta, recipient of almost every award that economists can bestow, has pointed out that most of the recent GNP increases of 2nd/3rd world countries have come at the expense of their average citizens’ personal assets (Dasgupta 2003).Africa’s burgeoning population exacerbates all of its problems. As of 2015, the UN’s mid-range population growth projection predicts that it will have ~4.5 billion inhabitants by 2100 AD – about three times that similarly anticipated for the world’s currently most populous nation, China. The populations of 28 African countries are supposed to more than double between 2015 and 2050 and, by 2100, those of Angola, Burundi, Democratic Republic of Congo, Malawi, Mali, Niger, Somalia, Uganda, United Republic of Tanzania and Zambia are to increase at least five-fold.Frankly, I consider these projections unrealistic. Both the Western world’s current political trends (widespread “populism” (polarization) driven primarily by increasing class, power, and wealth disparities) and most of human history suggest that another “world war” is apt to kill far more people than did the 20th century’s and thereby curb populations, especially within poorer counties. US Pentagon studies (CAN 2014) concluded that the majority of such deaths will be due to disease and starvation caused by the disintegration of technology-dependent societies contending for increasingly limited and often degraded resources (land, food, fuel, high grade ores, etc.). In his book, "Small is Beautiful: A Study of Economics as if People Mattered", Ernst Schumacher (Schumacher 1973) observed that today’s technological civilization is unsustainable because the finite resources enabling it are treated as inventory (income) rather than capital. The “sustainability” of today’s economic systems therefore requires continued growth of both population and total wealth (GDP) which is impossible in a finite world. Consequently our goal should be a genuinely sustainable and much more egalitarian economy in which every individual regardless of where they live or who they know does indeed matter. Until we acknowledge those facts, accept that goal, and begin to act accordingly, we're just spinning our wheels.Since food represents humanity’s most basic need and its source is farmland, I’ll begin by describing what’s been happening along those lines in Africa. A recent Brookings Institute report (McArthur 2013), points out that, “no matter how effectively other conditions are remedied, per capita food production in Africa will continue to decrease unless soil fertility depletion is effectively addressed.” It goes on to say that a second major problem with the oft-assumed African “land abundance” hypothesis is its inconsistency with evidence that its soils are being simultaneously depleted and eroded by current agricultural practices including a decline in fallowing. While some African leaders along with the management of “land grabbing” (?) international agribusiness concerns seem to feel that Africa still has plenty of yet-undeveloped arable land, many of Africa’s poorest people (mostly subsistence farmers) can’t afford to let any of theirs lie fallow: some families live on 0.9 US acre (not ha) farms yielding under 1 t of grain/ha while the first-world’s farmers routinely produce 3 to12 t/ha of whatever “cash crop” they chose to plant on several order of magnitude larger farms.The key differences between the agricultural practices of developed nations and most of Africa’s include:1.Developed nations heavily fertilize their croplands – most of Africa’s farmers can’t afford artificial fertilizers and often have to burn any manures or crop residues they can gather to cook their food.2.Developed nations’ farmers can afford to irrigate their croplands – most of Africa’s can’t, which issue is compounded by the fact that much of its nominally arable land doesn’t get enough rain to reliably support anything other than skeletal cows or goats.3.Most developed-nation farms are large and productive enough to enable their owners to buy/utilize specialized machinery which renders their labor both far less exhausting and much more rewarding. The world’s poorest farmers work themselves to death with primitive tools.Why Everything Boils Down to Energy InputsOf course, those differences simply reflect the relative amounts of raw/primary energy supporting the lifestyles of rich vs. poor people, which measure in today’s world boils down to their relative per capita fossil fuel consumptions. On-farm agricultural energy consumption in rich countries entails the burning of diesel oil, gasoline, and/or LP gas in engines plus use of electricity generally made by burning more fossil fuel, usually coal. It is considerably higher in high-GDP countries (around 20.4 GJ/ha) than it is in low-GDP countries (around 11.1 GJ/ha) and far greater than on Africa’s poorer subsistence farms (Giampietro 2002). For example, most of the energy input to a subsistence farm consists of human labor which, throughout an 8 hour work day, amounts to about 75 watts per person (Human Power 2018). If 100% of such useful (mechanical) energy (75*8*3600 = 2.16E+6 J/day) is devoted to cultivating a 0.9 acre (0.36 ha) plot throughout a 6 month growing season, the area-normalized “energy services” devoted to that effort is 1.08 GJ/ha/a (2.16E+6 J/day*365days*6/12/2.47 acre/ha/1E+9) which is about ten percent of the raw/primary food energy required to keep each person so-occupied alive throughout the year (2500 kcal/day *365 days/a). Energy-wise that’s not very efficient – state of the art farm machinery generates about one third of a joule’s worth of useful mechanical energy from a joule’s worth of fuel heat energy and don’t consume anything when not running.According to a “Food and Agriculture Organization of the United Nations” (FAO) report (Sims 2011), the raw/primary energy consumed by the world’s “food sector” amounts to ~95 exa (1018) Joules – approximately 20 percent of current total global raw/primary energy consumption (~ 570 EJ exa (1018) Joules) – and generates over 20 percent of anthropogenic greenhouse gas emissions. Land use changes, particularly those linked to the deforestation brought about by the expansion of agricultural lands to raise food crops and biofuels (IPCC 2007) constitutes another ~15 percent of anthropogenic GHG emissions. Only about 5% of that energy, ~6 EJ, directly supports on-farm activities such as cultivating and harvesting crops, pumping water, housing livestock, heating protected crops, drying and, short term storage. The rest/majority of the agricultural sector’s energy demand is devoted to transport, fertilizer and pesticide production, food processing, packaging, storage, and distribution.All of the world’s developed countries have adopted Dr. Borlaug’s fossil-fueled “Green Revolution” which enabled ~90% of the world’s ~7.5 billion people to consume as much food – both basic necessities along with some luxury items – as they want. Approximately one half of the world’s current population would quickly starve if that hadn’t happened. However, the fossil fuels enabling both that revolution and most of the 20th century’s other ones generated environmental impacts including the greenhouse gas (GHG) emissions responsible for global warming/climate change. From 1870 to the present, fossil fuel burning dumped about 580 Gt (580 Pg C) into the atmosphere in the form of ~2100 Gt of CO2. That gas partitioned between the atmosphere, oceans and land, warming all of them & thereby causing increasingly severe and frequent weather events including “Super El Niños” (Hong 2016), ocean acidification, drought and biofuel production-driven food cost escalation, air pollution, deforestation, potable/irrigation water shortages, sea-shoreline erosion/flooding, and relentless cost of living increases in the world’s poorer regions. Those effects constitute threat multipliers that aggravated stressors - poverty, environmental degradation, hunger, political instability, and social tensions – that engendered mass migrations as well as terrorist activity and other forms of violence. During that same ~150 years, two world wars and numerous smaller ones were fought over access to natural resources – primarily “lebensraum” (land) and fossil energy, usually petroleum – some of which resulted in the Christian-country “winners” creating new countries in the oil rich Islamic Persian Gulf, which, of course, eventually engendered more conflict.Securing those resources has proven to be expensive to those war’s winners. A Princeton University report concluded that simply keeping the US Navy’s fifth fleet within the Persian gulf from 1976 to 2006 had cost its taxpayers ~$6.8 trillion (2008 dollars) and would probably cost them another $0.5 trillion during 2007 (Stern 2010) which figures didn’t include the costs of actual conflicts. Since that fleet remains on station, the total cost of “maintaining presence” therein has now probably reached about $12 trillion. A 2013 Kennedy School of Government report (Foreignpolicy 2013) concluded that the total cost of the USA’s most recent wars in the Middle East and Northern Africa would probably be $4-6 trillion and had accounted for roughly 20 percent of its increase in national debt between 2001 and 2012 (modern wars are fought with borrowed money).Concerted international effort to address fossil fuel’s environmental impacts began with the UN’s 1997 Kyoto Protocol to which many, mostly small & not particularly impactful, countries signed up. While the billions of dollars spent on climate science research since then have generated thousands of papers/reports and paid for hundreds of other conferences both large and small, neither that science/knowledge nor the policy changes implemented by many countries which favor/subsidize politically correct renewable energy sources have had much effect upon mankind’s GHG emissions. As this is being written (December 2018) representatives from 195 countries have again gathered (in Katowice, Poland) for this year’s United Nations Climate Change Conference, COP 24 (COP = Conference of the Parties). This meeting is focused upon producing rules to flesh out the “details” of the 2015 Paris climate accord (COP 21), the landmark agreement signed by all of its attendees except Nicaragua and Syria, to battle climate change and hopefully limit global warming to <1.5 degree Celsius, one-half degree under the 2°C limit set earlier at COP 15 (Copenhagen conference). Since 2015, the International Panel on Climate Change’s (IPCC’s) leadership has been trying to breathe new life into that accord amid backsliding from several key nations, most notably the United States over commitments made when they signed it. To date, the IPCC’s efforts have not really accomplished much because key “parties” refuse to agree upon a mechanism ensuring that they honor their commitments with respect to either GHG emissions or contributions to a $100 billion/a climate mitigation fund.The latest version of the British Petroleum company’s annual Statistical Review of World Energy (BP 2018) contains not only information from the preceding year, but also historic data on consumption and production of all forms of energy during the last several decades. Its principal conclusion is that humanity is not fulfilling the goals set by the Paris Agreement regarding the threats posed by climate change. In 2017, Mankind took a step backwards with respect to timid advances made during the two preceding years: the use of fossil fuels had grown increasing CO2 emissions by ~1.6%. Apparently that trend continues – anthropogenic CO2 emissions rose another 2.7% in 2018 (Jackson et al, 2018). Worse, most climate models indicate that by 2100 AD, even if the “commitments” made by COP 21-24’s attendees were to be honored, in toto they would likely cause global warming of between 2.7 and 3.2 degrees Celsius, well above the 1.5- 2 degree threshold that many climate modeling experts consider a tipping point beyond which Nature’s positive feedback mechanisms will render catastrophic impact inevitable (Hansen 2008, Hansen 2016).The most important thing mankind must do is to replace today’s fossil fuel dominated energy system with something that is both “clean” (no GHG gas emissions) and sufficiently reliable (not “intermittent’) to power an even bigger, more interconnected, more prosperous, cleaner and fairer future world economy.How much such energy would be needed?The exceptionally “rich” lifestyle of the USA’s ~320 million people is supported by about 99.5 EJ (98 quads) of raw/primary energy per annum, ~80 percent of which is provided by fossil fuels (LLNL 2015). That translates to a mean per capita raw/primary energy consumption rate (power) of 9860 watts (99.5E+18J/3600/24/365/320E+6) or about eight (99.5/3.2e+8/570/7.5e+9) times that of the world’s average person today. Since one joule’s worth of raw/primary (heat) energy provides about 0.4 joules worth of useful “energy services” (the efficiency of most of fossil fuel’s applications is Carnot-limited) and Europeans apparently can live almost as well consuming one-half that much raw/primary energy per capita, let’s assume that supporting the life styles of each of the future’s equally EU-rich/happy people would require ~2 (9860* 0.5*0.4 = 1972 ≈ 2000) kW’s worth of energy services (electricity). Consequently, a world with 11.2 billion such inhabitants must possess power plants able to supply an average power of about 22 (11.2E+9*2000*3600*24*365/1E+12/3600/24/365 = 22.4) TWe (terawatt electrical). Finally, assuming that each individual region’s peak power demand is ~40% higher than its average and that no world-wide, zero-loss, “super grid” exists, our descendants would need ~30,000 (22.4*1.4*1012/109) one GWe power plants to live that well.That power could not be generated with fossil fuel because even if there were enough of it (there isn’t), burning it would have catastrophic consequences. For example, the raw/primary (heat) energy represented by the world’s remaining 1139 billion tonnes of coal, 187 trillion m3 of natural gas, and 1.707 trillion barrels of petroleum, (BP 2017) is about 5.0E+22 J’s which, if consumed by 40% Carnot efficient power plants, could generate 22 TWe for just 29 years - ~⅓ of a typical first-world human life span. Additionally, those fuel reserves collectively contain about 1200 Gt of carbon which, if converted to CO2 and dumped into the atmosphere would push global warming well past any of the tipping points suggested by the world’s climate modeling experts.Because the “half life” of CO2 already in the atmosphere is about a half century (Moore and Braswell 1994), achieving the goals of the Paris climate accord (limiting maximum temperature rise to 1.5C) at this late point in time would require an almost immediate switch to clean (no GHG emissions) energy sources and the removal of enough carbon dioxide already in the atmosphere to reduce its concentration to ~350 ppm by volume (Hansen 2008). Consequently, some of the ICPP’s more optimistic post-COP 20 scenarios/reports assume that “bio-energy with carbon capture and storage” (BECCS) represents a magic bullet that could address both global warming and the future’s energy supply conundrum in a politically correct (no nuclear) fashion (Martin 2016). All such scenarios are unrealistic because raising sufficient switch grass, palm oil, wood, etc. to do so would require vast amounts of land, water, & fertilizer that most people (especially the hungrier ones) would consider better-utilized if applied to producing something rather other than fuel. It is also unrealistic because carbon capture and sequestration (CCS) is both difficult and expensive which is why after several decades and many billions of dollars-worth of “study” and “demonstrations”, only about 0.08% of anthropogenic CO2 is currently so-managed (CCS 2018). BECCS-based “save the world” scenarios are also impossible because they don’t scale. For example, burning 100% of the world’s current annual grain (about 2.5 Gt, see Statista 2017) plus “bone dry wood” (about 1.9 Gt, see Wood 2018) harvests in “clean” (CCS equipped) 40% efficient (optimistic) heat-to-electricity power plants would generate useful energy services (electricity) equivalent to the output of ~935 one GWe (“full sized”) nuclear reactors –~3% of the number required to render 11.2 billion people one-half as energy rich as the USA’s are now. Any backup system for low capacity factor energy sources (solar and wind) must be able to satisfy most, not just 3%, of total demand. Furthermore, the carbon (about 1.8 Gt) in that much biofuel (~carbohydrate) represents only about 0.3% of mankind’s total anthropogenic carbon emissions to date which means that even if 100% of the CO2 it represents were to be captured and sequestered, it wouldn’t actually accomplish much. Consequently, because it could not achieve either of the IPCC’s goals and would surely compete with food, fiber, and construction-type wood production, all rosy BECCS-based scenarios are hopelessly unrealistic and therefore do not “deserve further study” - the conclusion common to the majority of reports in any scientific field. Additionally, because growing biofuel removes inorganic nutrients and soil organic carbon, it’s just another extractive technology that would further degrade the environment while compromising food production (Lal 2008).The most alarming thing about how things have been going recently (see the Fourth National Climate Assessment - NCA4 2018) is that civilization remains absolutely dependent upon resources that will inevitably become prohibitively expensive when most of the cheaper/easier-to-access coal, oil, and natural gas has been consumed which situation is likely to occur well before 2100 AD. Unless the world’s decision makers have already developed/implemented a simultaneously “clean”, reliable (not intermittent), and affordable alternative by then, civilization is apt to collapse heralding the onset of a dark ages akin to that depicted in Mad Max movies.This “Technological Fix’s” SpecificsLet’s do some ball park calculations that demonstrate how Weinberg and Goeller’s vision could address Africa’s (and the world’s) technical issues.AssumptionsFirst, I’m (reluctantly) going to assume that the UN’s population projection for Africa circa 2100 (about 4.5 billion) turns out to be right.Next, since I’m primarily concerned with showing what a nuclear renaissance should be able to accomplish with respect to assuring that continent’s (and the world’s) food security, I’m going to assume that part of the useful energy (electricity) it provides is devoted to doing so – in other words, nuclear power would provide the necessary water, fertilizer, and soil-building minerals plus that required to properly utilize them.Finally, I’m going to assume that Africa’s decision makers along with who/whatever else chooses to help/enable them, collectively decide that its citizens should be able to enjoy the same standard of living enjoyed by the EU’s today.Why Politically Correct Renewables Couldn’t Save the WorldAssuming that “energy services” means electricity (reasonable because most of its applications are nearly 100% efficient) and that no worldwide power grid exists circa 2100, generating Africa’s share of the world’s total energy supply would require about 12,000 (30*4.5/11.2*1012/109) full-sized (~one GWe) power plants generating an average of 9 TWe (4.5E+9*2 kW).The following shows why today’s most popular alternative/renewable energy sources could not meet that demand.First, let’s see how many of today’s purportedly “cheap”, roof top-type, solar panels would be required to produce 9 TWe’s worth of useful energy. At Home Depot today one can purchase four, real state of the art (19% efficient), 265 watt-rated, 1.61 m3 (39” by 65”) solar panels for $1412. If they were to be employed in Nigeria which purportedly exhibits an average solar irradiance of 5.5 kWh/m2/day (Ojuso 1990), each of those panels would generate a time-averaged power of 70.1 Watts (1.61*0.19*5500*3600/(24*3600) ) which means that their “capacity factor” (average energy/power generated/nameplate rating) when so-situated would be 26.4% (70.1/265) – about two and one-half times what it would be if installed in northern Europe instead. This suggests that powering Africa’s 4.5 billion future citizens with them would require 128 billion (900E+9/70.1) such panels costing about $45 trillion of today’s dollars. Since such power is intermittent, they would also have to buy enough batteries to keep everything running during the roughly 73.6% (100-26.4) of the time that their panels would not be producing much. How many batteries would that be? Assuming that Africa’s inhabitants could get by with just 1 day’s-worth of energy storage (unconservative - widespread cloudy and windless periods often last longer than one day) they would have to build/buy about 200 billion kWh worth of storage capacity (2000 J/s*4.5E+9*3600 s/hr*24 hr/day/3.6 E6 J/kWh = 2.16E+11≈ 200 billion kWh), which, if implemented with Tesla's equally real and state-of-the-art 13.5 kWh, ~$7000, lithium ion battery "Power Walls", would cost today's subsistence farmers’ hopefully more prosperous descendants another $100 trillion ($7000/13 kWh*200E+9 kWh/3.6E+6 J/kWh) of today’s dollars to purchase the first time (batteries only last for a few years). Real world windmills exhibit similar capacity factors meaning that if they were to be used instead, a similar amount of energy storage capacity or some other sort of clean backup power would be required. Natural gas – today’s primary backup for (& thereby justification for financial investment in (Buffet 2014)) today’s heavily subsidized wind & solar power plants – will probably be prohibitively expensive by then because all of the “cheap” natural gas will have already been fracked/consumed. Leaving it in the ground along with most of the world’s remaining coal would be a good idea because burning them would otherwise add to the already excessive amounts of anthropogenic greenhouse gases (GHGs) responsible for climate change – coal as CO2 and methane both as-is and after it’s been oxidized to CO2 - methane is a far “stronger” GHG than is CO2 and several percent of that currently fracked leaks directly into the atmosphere (Alvarez 2012).Which Crops and How Much Land?Next, we must decide what crops Africa’s future people should grow and how much land would be required to do it. For simplicity’s sake, let’s assume that the everyone will be consuming 2500 kcal/day (1.05E+7 J) of food, most of which would be provided by two especially productive crops raised upon the minimum amount of soil capable of providing yields currently achieved in the USA.Since I can’t cut and paste WORD/EXCEL tables into QUORA & there doesn’t seem to be an easy way to do them from scratch, I’ll have to summarize this section. Data from Clark and Tillman’s 2017 paper discussing the amount of land (m2) a produce a gram of proteins with different crops, USDA data (http://usda.mannlib.cornell.edu/usda/current/Acre/Acre-06-29-2018.pdf ) on US crop yields/acre, and candidate food crop characteristics from WIKIPEDIA entries suggests that an efficient combination would comprise maize (corn) because it’s exceptionally productive, nutritious, and already widely produced/consumed/accepted in Africa plus some sort of pulse (legume) to complement its unbalanced mix of amino acids (not enough lysine) (Lal 2017). Of the likely pulses, peanuts seem to make the most sense because they are a “hot weather crop”, taste considerably better, contain more fat/oil, also already widely produced/consumed/accepted in Africa, and would not extract as much phosphorous and potassium (key macronutrients) from its soil per food-calorie as would the next runner-up, soybeans.Assuming zero waste, providing 2500 kcal/day of food for 4.5 billion people translates to 4.1E+15 kcal (1.72E+19 J) worth of foodstuffs per year. If we also assume that 75% of their food-type calories are to be provided by maize, simple calculations indicate that the total amount of land required to feed every African circa 2100 AD adds up to 9.71E+7 ha, of which 7.28E+7 ha would be devoted to maize and 2.43E+7 ha to peanutsThat combination would provide everyone with 59 grams of “complete” protein per day along with virtually everything else that humans need to first grow and then remain healthy. Africa’s folks would probably also want to (and should) devote perhaps an additional 5-10% of similarly productive/managed land to raising the lower calorie/protein but tastier fruits, vegetables, and spices that render vegetarian diets far more palatable than most people realize. Additionally, if Africa’s much more prosperous (on the average) future inhabitants were to decide that chicken should provide 20% of their food calories (500 kcal/day/person – about fourteen times more than they currently consume), similar calculations suggest that roughly 10% additional land would be required to raise the peanuts and maize needed to feed those birds too. The substitution of cricket “meat” (Van Huis 2012) for that much chicken would require only about 5% more peanuts/corn/land than would a strictly vegetarian dietary.9.71E+7 ha is about the same amount of land that the USA currently devotes to producing all of the crops listed in Clark and Tillman’s paper to support its ~320 million people (7.2% of the number assumed herein for Africa). Since Africa has a total area of 29.6E+8 ha, 7.4% of which is purportedly arable (Worldstat 2018), 9.71E+7 ha represents only 44% of its arable land which, hopefully, means that Africa’s future citizens would choose to continue to share some of their still useful land with its iconic suite of wild animals.The Whys and Costs of DesalinationAfrica’s 4.5 billion future inhabitants wouldn’t be able to feed themselves with ~50% of its arable land unless they become able to irrigate it. Because irrigated land almost always produces higher yields than do rain fed farms and permits double and sometimes even triple cropping in warmer regions, such lands currently provide around 40% percent of global cereal supply (FAO 2011a). Only ~4% percent of Africa’s cropland is currently irrigated due primarily to prohibitive costs, insufficient water, and a general lack of commitment to infrastructure-related investment in things like power plants & the fuel needed to operate them. Consequently, it’s unlikely that this essay’s cornucopian scenario would be implemented by either Africans themselves or the institutions/people that have provided most of their “aid” to date.Pumping water onto approximately 10 percent of the world’s total arable land (around 300 Mha) currently consumes around 0.225 EJ/yr. Another 0.05 EJ/yr of indirect energy is devoted to the manufacture and delivery of irrigation equipment (Smil 2008). Around two-thirds of the water currently used for irrigation is drawn from underground aquifers. Energy intensive electricity powered deep well pumping accounts for about two-thirds of that and projections suggest that it will become ~90% by 2050 when shallow reserves are almost totally depleted. Current aquifer water extraction rates exceed recharge rates– grossly so in many places. Additionally, global warming is simultaneously exacerbating droughts and melting the glaciers that feed the riverine systems providing much of the world’s cheap-to-deliver irrigation water. Global warming has caused Mount Kilimanjaro’s “snows” to disappear along with most of those within the USA’s Glacier National Park. Building more dams won’t solve these problems because they don’t create water. Additionally, a comprehensive review of Nigeria’s outside-funded dam projects (Tomlinson 2018) concluded that while they do make money for local promoters and the outsiders that fund/support them, they decrease net agricultural productivity by turning fertile downstream floodplains into deserts. In addition to killing wetland-dependent wildlife, those dams have served to lower not raise, the incomes of far more people than have benefitted. Most such dams don’t generate nearly as much electrical power as “promised” due to inadequate maintenance and low (water-limited) capacity factors. Finally, at best, dams represent a temporary fix for the problems that they are supposed to address because their reservoirs eventually fill with mud.Water shortages plus the high cost of desalination – primarily due to high electricity costs – has led some countries rich enough to do so (e.g., China) to reduce their own crop production and rely more heavily upon imported grains.This situation is unsustainable which means that we’ll next assume that most of the water irrigating Africa’s future farmlands would be generated by desalinating seawater – the world’s only truly inexhaustible/sustainable water source. Wikipedia’s description of Israel’s solution to its water issues (Israel 2018), demonstrates how a properly managed future technological society could address the whole world’s water-related woes. Israel’s ~8.5 million people are fed by ~1.045E+9 m3 of fresh water applied to its mostly-irrigated farmland (Jewish 2016). This suggests that the rest of the even more water-stressed Middle East’s mostly-poor ~101 million people, including the majority of those living in Palestine, Iraq, Jordan, Lebanon, Oman, Syria, and Yemen (Demographics 2018), could be equally well supported by irrigating their potentially arable land (assuming all of it) with 1.24E+10 m3 of desalinated seawater. Assuming the ~3kWh/m3 energy requirement (RO 2018) of today’s most popular approach to desalination, reverse osmosis (RO), doing so would require an energy input of 1.34E+17 joules/a, which corresponds to the full-time output of ~4.2 one-GWe nuclear reactors. The volume of water corresponding to adding 0.51 meter (20”) of it over 9.71E+7 ha of African farmland is 4.93E+11 m3, which, if generated via RO, would require the full-time output of ~169 full-sized nuclear reactors (5.33E+18 Je/a). In principle at least, Siemen’s electro dialysis-based desalination technology would require only about one-half that number of nuclear reactors and is also less apt to become fouled by seawater’s other-than-salt impurities (Hussain, & Abolaban 2014).To continue, Africa’s average elevation is about 600 m (2,000 ft) above sea level, roughly the same as that of both North and South America. Assuming that all of Africa’s desalinated irrigation water would have to be pumped uphill that far, the energy needed to do so would be 2.90E+18 joules (4.97E+11m3*1000 kg/m3* 600 m*9.8 m/s) requiring another 92 full-sized power plants.How much would Africa’s desalination equipment cost? The contractual cost of the world’s (Saudi Arabia’s) biggest (~one million m3/day), RO-based desalination plant is $1.89 billion (Desalination 2018). Collectively, these numbers suggest that building enough RO plants to irrigate Africa’s future farmlands would require a one-time capital expenditure of $2.55 trillion (4.93E+11*0.00189/(365*1E+6) – about 11% of the USA’s current national debt. (Similarly addressing California’s Central Valley’s chronic irrigation water problems should cost only about $40 billion).As mentioned earlier, the Western World’s recent Middle East military incursions will probably end up costing its people ~thirty times more (4-6 $trillion) than it would to build enough nuclear powered desalination plants to provide sufficient fresh water for everyone living there and thereby address a root cause of the that region’s turmoil. For example a recent paper in the Proceedings of the (US) National Academy of Sciences (Kelly et al, 2014 ) points out that the chief driver for today’s Syrian conflict is the unrest/poverty generated by relentlessly worsening droughts, not a desire for “regime change” .Another plus for desalination is that its product does not add additional salts to soil and is also better at remediating already over-salinized soils than is ground water. A final plus is that because it doesn’t already contain near-equilibrium levels of calcium, magnesium, carbonate/bicarbonate, silica, etc., it is a better rock solvent (more corrosive) than is ground water. The next section will discuss why this is important.FertilizersOne of the reasons why the productivity of Africa’s farmlands is lower than that of more developed regions is that relatively little fertilizer is used. The three most important components of fertilizers (macronutrients) include nitrogen in either its negative three (ammonia-type) or positive five (nitrate-type) oxidation states, potassium (invariably in its plus one oxidation state), and phosphorous (invariably in its plus five oxidation state). Nitrogen fertilizer production currently accounts for about one half of the fossil fuel (mostly natural gas) used in primary food production.Nitrogen and the Cost of Fixing ItWe’ll start with nitrogen. US farmers hoping to produce 13.1 Mg/ha (200 bu/acre) of maize (grain) are advised to add ~ 258 kg of N/ha (PSU 2018). Since peanuts (a legume), can recover/fix its own nitrogen from air, much less nitrogenous fertilizer would be needed for land devoted to its cultivation – let’s say 50 kg N/ha. Assuming those application rates, fertilizing Africa’s future crop land would require ~ 2.0E+7 tonnes of ammonia each year (one kg N ≈ 1.21 kg of ammonia) A recent review of synthesis technologies (Frattini 2016) concludes that each tonne of ammonia made with electrochemically-generated hydrogen, pressure swing-generated atmospheric nitrogen, and conventional Haber Bosch processing equipment would require about 14.2 MWh’s (14.2*3.6E+9 J) worth of electricity. That, in turn, suggests that satisfying this chapter’s scenario’s nitrogenous fertilizer requirement would require the full-time output of ~32 one-GWe power plants.The Reasons Why Basalt Should Supply P&KSince….Much of the Africa’s (and the world’s) farmland has already lost a good deal of its topsoil via erosion·Much of its remaining topsoil is mineral-depleted·Basic (mafic) rock-weathering is how Mother Nature limits the Earth’s atmospheric CO2 concentration (Hartmann 2013)·Basaltic rocks are both intrinsically basic (contain a good deal of magnesium and calcium) and rapidly weathered by the natural phenomena extant in cultivated soils (Moulton 2000)·Most of the Earth’s crust consists of basaltic rock, a good deal of which is either on or close to the surface of its continents·Basaltic rocks contain relatively high concentrations of potassium and phosphorous along with all other biologically important elements, which is why…·…Soils comprised primarily of weathered volcanic ash most of which originally consisted of molten basalt are exceptionally productive (Beerling 2018)…we’ll next assume that the phosphorous and potassium required to produce Africa’s food crops circa 2100 AD will be provided by amending its farmland with powdered basalt. In order to be effective any such amendment must weather rapidly enough to release sufficient potassium and phosphorous to support high-yield agriculture which, in turn, means that the raw rock surfaces must be “fresh” (not already equilibrated with the atmosphere and thereby covered with secondary phases), ground to a considerably smaller particle size than is the quarry waste-type soil amendment rock powder currently being marketed to hobby farmers, and mixed with root-zone topsoil, not just dumped upon the surface of the ground (Campbell 2009 and Priyono/Gilkes 2004). Based upon the rather limited amount of scientifically planned/supervised experimentation in open-access (not paywalled) technical literature, I’m going to assume that this would require grinding it so that the particles comprising >80% of its mass possess diameters <10 microns. Since rock grinding is highly energy intensive – much more so than is simply recovering it from a quarry’s rock outcrop or waste dump – the cost estimate for this part of my scenario will be based upon that step’s energy demand plus the powder’s transport and distribution costs.First, how much of it must be made? The food stuff P and K concentrations, land areas, and crop yield figures in Tables 1-3, suggest that the food consumed each year by Africa’s 4.5 billion future inhabitants would contain 2.64E+9 kg of potassium and 2.05E+9 kg of phosphorous. Assuming (wrongly I hope, but consistent with the way that things are usually done) that neither nutrient is subsequently recycled back to the soil, both must be replaced each year via basalt weathering. The compositions of flood basalts vary considerably but since all originate from the Earth’s fairly well mixed underlying magma, for the following estimates I’m going to assume a composition with which I’m familiar (Leeman 1982 and Siemer 2019) – that of the basalt comprising Idaho’s “Craters of the Moon” National Monument and also covering much of the rest of Idaho’s Snake River Plain. It contains an average of 0.61 wt% K2O and 0.55 wt% P2O5 which translates to requiring 5.21E+8 Mg (tonnes) of it per year to provide this scenario’s potassium and 8.69E+8 Mg to supply its phosphorous. Since phosphorous happens to be the limiting nutrient in this case, at steady-state, we’d be adding 8.95 Mg (8.69E+8/9.71E+7) of powdered basalt/ha/a.A recent review of rock grinding technologies (Jankovic 2003) suggests that producing one Mg of <10 micron basalt powder would require about 100 kWh’s worth of electricity. If so, making 8.69E+8 Mg of it would require 3.13E+17 J, which if done throughout one year would require the full-time output of 9.9 one-GWe nuclear reactors.If this powder were to be transported an average of 1930 km (1200 miles) from mine-to-farm via an electrified rail system as energy-efficient as that currently used to move US coal (185 km/L diesel fuel/short ton), its energy cost would be about 6.80+16 J (assumes 1.1 Mg/short ton, 33% heat-to-mechanical engine efficiency and 44.5 MJ/kg diesel fuel with a SpG of 0.85). Trebling that figure to account for fuel consumed by trucks and tractors at the rail heads, brings the total to 2.04E+17 joules/a which corresponds to an annual transportation/distribution energy demand requiring another 6.5 one-GWe nuclear reactors to satisfy.An application rate of 8.95 Mg/ha/a is not really very large because it represents only about 0.5% of the mineral matter already in a six inch deep (root zone) layer of normal density/composition soil and is also considerably less than what current farming practices lose via wind/water erosion (typically about 30 t/ha/a - Pimental 2009). Consequently, since this scenario’s rock grinding/distribution costs are much lower than its irrigation water and nitrogenous fertilizer costs, it would be a good idea to at least start out with considerably larger application rates, perhaps 40-50 Mg/ha. Doing so would also reduce the chance of crops being “starved” due to slower-than-I’ve-assumed weathering rates.The 309 (169+92+32+9.9+6.5) GWe’s worth of “clean” power plants required to implement the agricultural aspects of Africa’s clean/green future is about the same amount of power currently generated by all of the world’s nuclear reactors and over a hundred times more than Africa currently produces in any fashion (about 650 TWh, see Energy in Africa 2018). However, it represents only ~3% of the total energy services required by 4.5 billion as rich as the EU’s are now..All of the necessarily huge machinery and manufacturing facilities required to implement this or any other technological fix capable of “saving the world” would be much cheaper to build and operate much more efficiently with reliable power than with that provided by intermittent sources. While it would indeed be “possible” to run desalination/ammonia plants, rock crushers, tractors, locomotives, etc., with windmills and/or solar panels, doing so would be expensive, dangerous, and frustratingly unproductive to such machinery’s owner-operators and their customers. Intermittent power supplies are suitable for some niche applications, not for powering technological civilizations (Brook 2018).Again, some of this section’s numbers are rough approximations because the rate and degree to which powdered basalt would release its constituents (weather) under field conditions is affected by a host of factors/variables. A nutrient-specific discussion of some of them may be found in a FAO report describing the use of raw phosphate rock as fertilizer (Zapata and Roy 2004). Thankfully, this subject is beginning to receive a good deal of attention (Taylor 2017) and some hopefully realistic studies have begun (Beerling 2018).Other “Killer Apps”Atmospheric carbon sequestrationWhen completely weathered by the mechanisms collectively responsible for doing so in biologically active soils, each gram of this scenario’s basis basalt (10.06 wt% CaO and 7.65 wt% MgO) would release 7.35 milliequivalents (0.1006*2/(40+16)+0.0765*2/(24.32+16)) worth of base. If we assume that it would convert soil-gas CO2 that would otherwise transpire (to the atmosphere) to bicarbonate ion (Hartmann 2013), the application/weathering of 8.95 t/ha of it over 9.71E+7 ha of African farmland would remove/sequester 0.076 Pg (76 million tonnes) of carbon. That sounds like a lot of “sequestration” but represents only ~ 0.009% of that currently in the atmosphere (~ 3300 Gt CO2).If carbon sequestration is to be a primary goal, another way to go about doing it would be convert corn stover (there’s about as much of it produced as grain and approximately 80% of it can be readily collected) and peanut hulls/stems (their leaves would probably end up on the ground) to “biochar”. Assuming this scenario’s African grain crops, that translates to converting about ~11.6 tonnes of biomass to ~3.1 tonnes of biochar and 5 tonnes of bio oil per ha (Extension 2002 and Fortress 2011). Because biochar is ~70% elemental carbon, burying it would simultaneously increase Africa’s soil’s organic matter (SOM) and sequester atmospheric carbon at the rate of ~0.25 Pg (250 million tonnes) per year. If everyone circa 2100AD –not just Africans - were to char their stover and fertilize with Snake River plain basalt, they would collectively sequester the equivalent of about 3 Gt CO2 per year. However, since the atmosphere already contains about 500 Gt of excess CO2 ((~412ppm-350ppm)/412ppm*3300 Gt =496) and will surely get further out of balance before we kick our addiction to fossil fuels, it would probably take over two centuries for the future’s farmers to reduce it to a “safe” (350 ppm) level.These numbers suggest that the primary rationale for implementing this particular “enhanced weathering” scheme (Hartmann 2014 & Beerling 2018) would be to stop polluting, make everyone “rich”, and render agriculture sustainable, not collect/sequester already-dumped atmospheric carbon.Motor/engine fuelsAnother rationale for “biocharing” is that it should simultaneously produce more than enough “oil” to fuel the machinery required to run the farms meaning that it could become a profitable side line for their owners. Figures in a recent report about Nebraska’s farm fuel costs suggest that high-input corn farming currently requires about 70 US gallons of diesel fuel/ha/a (Agecon 2015). Five tonnes of bio oil purportedly has the energy content of ~37% that much No. 2 diesel oil (i.e., 2176 liters, 575 US gallons, or 82 GJ’s worth of such fuel) and it should be possible to convert it to a good diesel-type engine fuel (Renato 2013).Another possibility raised by a suitably implemented nuclear renaissance is that other sorts of motor fuels could be synthesized because electrolytic hydrogen would become much cheaper than it is now. For instance, hydrogenation of the ~11.6 t/ha of stover mentioned previously would produce about 3x as much synthetic fuel oil (~4.9E+8 Mg/a) as would making it from biocharing’s bio oil (Agrawal 2007). Similarly, if Africa’s 4.5 billion future citizens were to produce/consume as much Portland cement per capita as we do now, Fisher Tropsch hydrogenation of the CO2 so-generated would produce about 8.2E+8 Mg of transportation fuel/a which figure divided by 4.5 billion represents ~28% of current world per capita petroleum consumption rate. Another possibility which would not serve to dump such carbon into the atmosphere would be to make additional ammonia and use it to fuel engines and/or fuel cells (Kanga and Holbrook 2015).MiscellaneousFinally, if Africa were to fully electrify itself via a properly implemented nuclear renaissance, its air and water would be cleaner, its homes and cities more livable, more and better (more interesting, better paying, and more secure ) employment opportunities would be available to its young people, population growth rate would drop precipitously (see CATO 2018 ) as would the degree of misery/desperation/frustration currently driving young people everywhere (mostly males) to join terrorist gangs and hate groups. In other words, Africa’s people would experience the same benefits of radically increased prosperity that So. Korea’s and China’s have recently enjoyed. An almost never mentioned (too politically incorrect) reason for China’s recent success is that its leadership adopted/enforced a one child per family policy at the same time they decided to encourage/enable its people to become entrepreneurial (Conly 2015). The purpose of that policy was to free up both time and capital which could then be (and was) devoted to “making China great”. It also rendered children born during that period especially “special” to both their parents and society at large which rendered their lives both more enjoyable and successful.The job of government is not just to give its current citizens anything they want, but to pave the way for a prosperous, stable society for their descendants. Any kind of government mandated fertility control is unattractive, but unless its goals can be achieved otherwise (by making the lives & futures of already living people better), it’s apt to become necessary everywhere.What sort of “Nuclear Renaissance” is needed?Regardless of how “small” and “modular” they might become, any “advanced” version of today’s light water reactors would consume at least ~160 tonnes of natural U/GWe /year, most of which, primarily 238U, would be discarded during its fuel fabrication’s U-enrichment step. A genuinely sustainable nuclear renaissance could not be implemented with them or any other sort of burner/converter-type reactor because the uranium industry's estimate of all “affordable proven plus undiscovered uranium resources” likely to be to be worth mining (in that context) is only ~18 million tonnes (Redbook 2014). Assuming 2 kW’s worth of LWR-generated electrical energy for 11.2 billion people, 100% of the world’s “affordable” uranium would be consumed within about 5 years (1.8E+7/ 22,000/160 = 5.1).People championing a burner/converter reactor-implemented nuclear renaissance do so based upon an economic argument rather than real geological/technical data; i.e., we are assured that sufficient uranium will inevitably be discovered when the definition of “affordability” rises beyond today’s upper limit, <,$260/ kg. For example, if a tenfold increase in price would indeed unearth 300 times as much uranium (Deffeyes & McGregor 1980), a LWR-based, 22 TWe, nuclear renaissance could be fueled for several centuries before the cost of its fuel (uranium) exceeded that of today’s coal-fired power plants. Unfortunately, there’s no proof that Mother Nature actually follows any such simple relationship which renders such assurances unconvincing (Höök &Tang 2013). For example, the uranium industry’s latest (2018) Redbook's figures do not support Deffeyes & McGregor’s rule-of-thumb; i.e., plots of its log10(resource size ) vs log10(cost) data possess slopes of from 1.10 to 1.25 depending upon whether it is an “identified”, “reasonably assured”, or “inferred” resource. McDeffeyes and McGregor’s rule of thumb implies a slope of 2.477 (log10 300/log10 10).Fueling burner/converter-type reactors with uranium extracted from seawater couldn’t “save the world” either. The country most involved with testing/developing that scenario is Japan (see Tamada 2009 for a slide set and lecture). Dr. Tamada’s slides begin with the contention usually prefacing such reports; i.e., that “there's 1000x as much U in the oceans as on land”. That’s incorrect because there's about 350 times as much U in “readily accessible” rock (the first kilometer of the ~3 ppm U crustal rock covering the earth’s land surfaces) as in its seawater (~1.33 billion km3 of ~3 ppb U water). Those slides go on to describe Japan’s pilot plant scale demonstrations and end with a conclusion that a 68.7 by 15.2 km (1030 km2) array of the most promising uranium adsorbent developed (amidioxime-coated, irradiated polyethylene fiber “ropes”) might be able to collect enough uranium to fuel six of Japan’s almost state-of-the-art LWRs (i.e., ~1200 Mg U/a) for a “reasonable” cost. That conclusion assumed that the adsorbent would be trapping ~4 g U/kg adsorbent per cycle, roughly three times more than was generally recovered during their demonstrations (Regalbuto 2014). A subsequent US study concluded that an “improved” version of that adsorbent would capture ~3.3 g U/kg adsorbent (Kim et al, 2014). In any case, because those adsorbent arrays must be bottom-anchored, experience significant wave action, and situated where natural currents quickly replenishes the water surrounding them, almost 4 million km2 of shallow (mostly coastal) ocean bottom would have to be covered to fuel 22 TW’s worth of conventional burner/converter-type reactors. Finally, anyone considering such schemes should be aware that both fishermen and “Rainbow Warriors” are apt to object - the former because their nets and lines would surely become entangled by so-situated gigantic synthetic “kelp beds“, the latter because those arrays might also entangle/strangle whales and turtles.Other than for those little details, mining the oceans to fuel conventional reactors is a really fine idea. On the other hand, Benjamin Bearman & Tony Chen, two of the folks who had read the more or less original version of this “answer” suggested that coupling Dr. Tamada’s uranium filters to a nuclear powered desalination plant would provide more than enough uranium to fuel a breeder-type reactor. They’re right, in principle at least, seawater so-filtered would contain about 20 times as much uranium than the‘reactor powering the desalination system would consume.Another common assertion, that the “reprocessing” of today’s accumulation of spent LWR fuel, could address the LWR fuel issue is equally silly. It takes about six spent LWR fuel assemblies to make one “MOX” fuel assembly and that process can only be done once. This means that the maximum possible such gain would be about 17%. MOX fuel is also expensive to make and relatively dangerous to use which is why most independent reviewers & all US electrical utilities have concluded that it’s not worth the effort. Reprocessing only makes sense if the recovered actinides go to fuel fast breeder-type reactors.Realization of Weinberg and Goeller’s utopian (but possible) future can happen only if the future’s decision makers decide to first develop and then implement an appropriately scaled (big enough) nuclear renaissance and then see to it that untrammeled human nature does not turn their project/vision into another nuclear boondoggle.A genuinely sustainable nuclear fuel cycle must be implemented with breeder-type reactors generating as least as much new fuel (“fissile”, any readily fissioned actinide isotope) as they consume from “fertile” natural uranium and/or thorium isotopes. Of the ways that that might be accomplished, fast spectrum molten salt reactors (MSRs) seem to offer the most promise (Holcombe Welcome!. 2016). One of the Generation IV International Forum’s (GIFs) Advanced Reactor (World Nuclear 2018) “Gen IV” concepts, EURATOM’s EVOL program’s “thorium burning” Molten Salt Fast Reactor (MSFR) is especially attractive (Fiorina 2013) . Siemer 2015 describes the features of an “isobreeding” (generates only as much new fissile (233U) from “fertile” 232Th as it “burns”, no extra) version of that concept. Isobreeding is an especially relevant goal/assumption because, at steady-state, the world would not require extra fissile which would mitigate proliferation concerns and it (isobreeding) would also simplify reactor operation because very little “reprocessing” would be required to keep it running at steady- state.Another power reactor concept which wasn’t formally considered by GIF, the Molten Chloride (salt) Fast Reactor (MCFR), would be simpler to build than the MSFR because its 238U -to- 239Pu “breeding” cycle’s superior neutronics should permit isobreeding without a fertile isotope (238U or 232Th) containing “blanket” surrounding its core. This would also mitigate proliferation concerns because at steady-state neither the reactor itself nor its attendant salt clean up/recycling system would contain “bomb grade” fissile (>90% 239Pu) that might otherwise tempt suicidal terrorists to attempt its “diversion”. Another of its practical advantages is that its core could be situated within a tank containing either a molten bismuth/lead “reflector” or a molten salt blanket containing a fertile isotope which would allow it to breed startup fissile for other reactors. These are probably some of the reasons why the Bill Gates-backed TerraPower “nuclear startup” recently decided to split its development efforts between its solid-fueled, liquid-metal cooled, “breed and burn”, “Traveling Wave” concept and an unblanketed, chloride salt-based “breed and burn” MSR (Southern 2018). Another startup, “Elysium”, is apparently proposing to build similar systems.Another especially promising MSR concept not considered by GIF is MOLTEX’s “stable salt reactor” (Moltex 2018). Its primary technical distinction is that its core consists of a bundle of thin-walled steel tubes containing a fissile isotope (any combination of trivalent 239Pu, 235U, and/or 233U) in a c molten chloride-based solvent salt consisting primarily of fertile 238UCl3 and table salt (NaCl). Unlike the others, its fuel salt would be “static”, not continuously recirculated between its core and external heat exchangers. Fission-generated heat energy would pass through the walls of those tubes to a rapidly moving (pumped) surrounding, fissile-free, fluoride-based coolant/blanket salt consisting of a ThF4 -NaF eutectic (Scott 2014). That molten salt stream’s heat energy would be transferred to a third molten salt stream (e.g., “solar salt”) which, in turn, would exchange its heat with water or CO2 to generate the pressurized gas driving its power turbines. Both the fuel and primary coolant salt streams would be rendered non-corrosive to conventional stainless steels via redox buffering with divalent Zr - an extremely powerful reducing agent/oxygen scavenger. In this writer’s opinion, the MOLTEX concept’s chief virtue is that it should be considerably easier/cheaper to implement the “first of a kind” version of it than either the MCFR or MSFR. Another significant plus is that its developers have been willing to reveal more “technical details” than have most of their competitors- credibility in this hyper secretive technical field is tough to earn and proportional to the degree to which a concept's champions embrace "openness".All of these concepts are “fast” because their cores do not contain a moderating material (e.g., liquid water, beryllium oxide, zirconium hydride, or elemental carbon) which deliberately slow the rapidly moving (fast) neutrons generated by nuclear fission. This enables superior fuel (fissile) regeneration capability, lessens minor actinide (Am, Cm, etc.) build-up, and permits operation with a much higher fission product “ash” salt concentration which, in turn, translates to a much lessened fuel salt reprocessing (clean up) requirement. It also means that they would not generate large amounts of solid waste comprised of irradiated/contaminated/damaged moderator. To date, the world’s graphite-moderated “production” (of weapons grade plutonium) reactors have generated roughly 250,000 tonnes of radiologically contaminated graphite, most of which lingers in “temporary” storage (TECDOC 2010). In principle, any of these reactor concepts could convert all actinides, both those introduced and generated in-situ, to relatively short-lived & simple-to-manage fission products (FP). All of them would obviate the cost, waste, and safety-related issues inherent to potentially sustainable, solid-fueled reactor concepts such as the sodium-cooled “Integral Fast Reactor” (IFR), “Traveling Wave” concepts or General Atomic’s helium-cooled “Energy Multiplier Module (EM2) (Rawls 2010). All of them should be cheaper to build than “advanced” versions of today’s ineluctably unsustainable light water reactors (LWRs) because they would operate at much lower/safer pressures and generate more useful (higher temperature) heat energy. And finally, all of them could be started with fuel comprised of the uranium, plutonium and minor actinides (TRU) extracted from spent LWR fuel assemblies which would simultaneously simplify/cheapen the long-term management of such “waste”.Since there isn’t enough 235U or spent LWR fuel-derived plus “excess” weapons-grade plutonium in the world (roughly 1900 tonnes total) to start more than ~400 of any sort of fast breeder reactor, they would have to be configured to breed extra start-up fissile until enough of them have been built to achieve steady state (power everything).All breeder reactor concepts save MOLTEX are at least 50 years old. None except the USA’s pet solid fueled, “liquid metal (cooled) fast breeder reactor” (LMFBR) technology ever received sufficient attention/funding to generate anything but “paper” (conceptual) reactors with, again, one exception – Dr. Weinberg’s/ORNL’s graphite moderated “molten salt reactor experiment” which ran for four years during the late 1960’s (MSRE 2018) but couldn’t “breed” because it was too small (8 MWt) and not surrounded with a blanket. The reason for this is that circa 1973, a financially strapped US federal government’s leadership (the Vietnam war had also been fought with borrowed money ballooning its national debt and triggering inflation) decided to fire the bothersome Dr. Weinberg (Weinberg 1994) and study only the LMFBR concept (it’s a much better plutonium maker) while its nuclear industry was selling much-enlarged versions of Admiral Rickover’s enriched-uranium-fueled, light water cooled/moderated submarine reactor at cost so that it could then profit by servicing (fueling) them thereafter. Although several large LMFBRs were subsequently built and operated by France and USSR/Russia, the concept never gained much traction with electrical utility owner/operators due to persistent sodium leaks/fires and the complex/expensive nature of both the reactors themselves (Mahaffey 2014) and the attendant solid fuel recycling systems that would have been required for sustainable operation. The main reason why genuinely sustainable nuclear fuel cycles still don’t get much attention in the US is that most of its political leaders consider nuclear power a stopgap while, with the “temporary” help of fracked natural gas, it “transitions” to an imaginary clean/green world powered by conservation, biofuels, wind turbines and solar panels/towers (plus super batteries?) linked together with a world-wide grid (Jacobson 2009, Jacobsen 2017). Consequently, the USA’s national laboratories’ nuclear scientists/engineers have helped their “industrial partners” render today’s unsustainable nuclear fuel cycle more attractive for temporary niche-filling by championing “small modular” versions of their current reactors even though it is unlikely that they would be as cost effective as were their full-sized forbears. Another reason why the development of a genuinely sustainable nuclear renaissance still gets short shrift is that doing so is impossible without first performing the “hot”, hands-on research (not “paper studies”) required to develop a practical/affordable system. 750-900 kg of fission products would be generated within any fast MSR’s fuel salt per GWe year all of which would have to be properly dealt with to prevent possible damage. Similarly, any tanks, pipes, and heat exchangers in contact with that salt would experience extremely high neutron bombardment rates which could also cause damage. Solutions to problems raised by these facts cannot be discovered until realistic tests are done under realistic conditions. Because the USA’s national laboratories have downsized their “hot” experimental facilities/capabilities and replaced them with “modelers”, it’s become extremely difficult/expensive to perform such work – most “research” money is currently spent upon buildings and personnel-related overhead.Finally, and most important, successful implementation of any of the concepts mentioned above could satisfy 100% of Mankind’s power needs with abundant & readily accessible natural actinide fuel - not just the 0.7% of natural uranium (235U) fissionable in a moderated reactor - which would render reliable/steady nuclear power as “renewable” as sunlight (Cohen 1983). For example, the earth’s crust contains an about 3 ppm U by weight. Some rocks (and all uranium ores) contain much more than that – average volcanic rock contains 20 -200 ppm, average black shale and phosphate rocks, 50—250 ppm (Ulmer-Scholle 2018). Assuming 200 Mev (3.2E-11 J) per fission, an average crustal rock density of 2.7 g/cc, and 50% heat to electricity conversion (molten salt reactors run much hotter than do LWRs and would therefore generate more electricity per heat joule), just the U within the topmost kilometer of the Earth’s continents (i.e., 3 ppm of ~4.2E+17 tonnes ≈ 1.2E+12 t U), could continuously generate 22 TWe for 74 million years. Since the earth’s crust contains 3 - 4 times as much thorium as uranium, let’s say a total of 12 ppm, breeder reactors could potentially generate ~2.7E+12 J’s worth of heat energy from one cubic meter of average crustal rock – about 56 times more than that provided by an equal volume of “banked” (in situ, not bulk) bituminous coal.Anyone who feels that such rock mining would be too impactful should consider:· the USA’s mountaintop-removal approach to coal mining· the fact that the fracking of the “easy” (shallow) 1.6 to 3.2 km deep US shale deposits (Lallanilla 2018) currently being mined, causes lots of mini earthquakes, occasionally pollutes ground water, and usually leaks some of its product directly into the atmosphere· the fact that Brazil’s much heralded recent offshore oil discoveries are considerably deeper yet and covered with both water and “rock” (Presalt 2018)· The fact that nuclear power is far less damaging to both the environment and people than is the coal industry (Kharecha, P.A., and J.E. Hansen, 2013)Reactor CostsIt is difficult to come up with definite cost figures in this particularly contentious arena because, like those of its health care system, there is little correlation between the USA’s “should” and “actual” reactors costs due to a tremendous burden of sometimes unnecessary, usually self-serving, and often litigious overhead costs. The effect of those cost drivers are best illustrated by a figure (Fig. 1) excerpted from a paper compiling historical construction costs for full-sized light water reactors throughout the world (Lovering, Yip, and Nordhaus 2016). The USA’s build cost per GWe started out at under one $billion 2010-dollars in the late 1960’s but quickly ballooned by over an order of magnitude (which eventually killed its nuclear industry) while those of more sternly disciplined countries (France and So Korea) have remained at about $2 billion for several decades.Figure 1: Historic worldwide inflation-adjusted power reactor build costsOver the long haul, any build cost under ~4 $billion/GWe is justifiable because the reactor’s product would be worth much more than that. For example, at 3 cents per kWh, the electricity generated by a one GWe reactor over 40 years would be worth $10.5 billion ($0.03/kWh*1E+9 J/s*3600 s/hr*24 hr/d*365 d/year*40 years/3.6E+6 J/kWh).Anyway, in 1970, a paper written by two of ORNL’s senior-most nuclear engineers (Bettis 1970) included an analysis of what power generated by a full-sized molten salt breeder reactor should cost. Their figure included the costs of construction ($0.159 billion 1970 dollars) fueling, and operating a one GWe breeder reactor. Applying the USA’s subsequent ~6.46 x inflation factor for most things to their conclusion ($0.0041/kWh), generates a should-cost figure of $0.0265/kWh – about 40% of the USA’s current average wholesale electricity cost. This suggests that if the USA’s ~320 million citizens set out to power themselves at the rate assumed herein for the future’s 11.2 billion people (2 kW average with a 40% peak) with one GWe molten salt breeder reactors, building the 857 (30000*0.32/11.2) of them so-required should cost about $860 billion of today’s dollars ( 857 *6.46 *$0.159 B), To put that figure into perspective, it is only about 35% (860/634=1.35) more than the USA’s current annual “discretionary” (nominally) military spending (OMB 2017). Similarly, since the USA’s national debt is now ~$21 trillion and the interest rate on its 10 year treasury bonds is ~3.19%, it seems likely that its taxpayers are paying someone (themselves?) ~$1.85 billion/day just to service their federal government’s debt, not address the issues threatening their children’s futures.Is $860 billion too much to simultaneously reestablish the USA’s “leadership in nuclear power”, clean up its atmosphere, restore/maintain the fertility of its farmland, and create millions of worthwhile (not just “service”) jobs and high-tech business opportunities for its citizens?Of course, since I’m pretending here that Africa’s population will be 4.5 billion, the cost of the ~12 thousand full sized reactors needed to power their part of world would be proportionately higher, ~$12 trillion of today’s dollars. To put that number into perspective, it’s ~12% of what just the backup batteries required by a similarly capable solar panel-based power supply system would cost. The reactors required to power only Africa’s future agricultural sector (feed them) in the manner described herein should cost ~$310 billion.How Could it Really Happen?It is unlikely that Goeller & Weinberg’s cornucopian scenario could be implemented by either the Africans themselves or those institutions/people that have provided most of their “aid” to date. Currently, all of mankind consumes ~2,300 GW’s worth of electrical power which averages out at about 307 watts/person. However, like most of the things that determine our life styles, its distribution is uneven. People in the Scandinavian countries consume the most, about 2500 watts per person, followed by the USA and Canada’s ~1400 W. However, the majority of Latin America’s consume under 250 W, South Asia’s below 100 W, and Africa’s under 25 W. Over a billion people now don’t have access to electricity at all. If mankind is to prosper, clean, affordable, and dependable (not intermittent) power must become available to everyone and it must be provided in a way that doesn’t pollute the air, poison the land, or change the climate. Avoiding a deadly “hothouse earth” will require the simultaneous redirection of humanity’s actions from selfish, for-profit exploitation to genuine stewardship and rapid transition to a genuinely sustainable economic system (Steffen 2018). It won’t be cheap or easy because rendering energy systems GHG free, ensuring that land, water and other resources are used sustainably, adapting to climate change and cleaning up an already polluted world will require substantial changes that many especially influential people and their vested interests will resist. It’s really a political, not technical problem and success will require project management like that of Admiral Rickover over 50 years ago - not management exhibiting the “symptoms” of most of the USA’s recent nuclear initiatives (Barriers to Science 1996).Goeller and Weinberg’s cornucopian future can’t happen if those most able to bear the costs of changing — those who’ve benefited the most from today’s economic system — don’t help to pay for it. Since interfering with current business practices is anathema to most of the Western world’s leadership, it’s likely that the East not the West will become the “outsider” helping the world’s disadvantaged people to implement this or any other scenario capable of achieving its goals. One reason for this is that most any of the West’s decision makers seem to believe that technical innovation in nuclear power should be left to the private sector, not government. While that might make sense for IT (hardware, software, and cell phone app development), it is unlikely to work because reactor development is much “riskier” requiring a far greater investment of money, time, special materials, experimental work, and experts able to address a host of tough mechanical, physical, chemical, and, especially, legal issues – it can’t be done by a few clever “hackers” in a rented garage. Its goals – achieving genuine sustainability, preventing further environmental damage, and providing a better life for everyone including the poor several decades from now – are also inconsistent with those of the majority of today’s venture capitalists. Getting government “out of the way” might sound nice but won’t work because every major energy breakthrough in recent history has received public support that moved an idea to proof-of-concept to demonstration after which the private sector heavily invested and thereby became richer (Siddiqui 2018).Of course, the other reason is that many of the West’s decision makers believe that the future should/could be powered by an “all of the above” mix of politically correct, renewable technologies (see Jacobsen 2009, Jacobsen 2017, and, for opposing views, Clack 2017 and Brook 2018) and have therefore supported/subsidized efforts consistent with that paradigm and, if not overtly hostile to anything “nuclear”, have paid only lip service to the development of a sustainable nuclear fuel cycle. This is understandable because the majority of US politicians were originally lawyers and/or businessmen (not engineers or scientists), and therefore don’t think quantitatively about technical issues – especially anything that’s “controversial” or apt to happen after their political tenure has ended.The most accurate measure of any country’s commitment to accomplishing anything is how much money it is willing to spend trying. In the world’s richest Western nation (USA ~ $20 trillion GDP) we hear that, “The US Department of Energy (DOE) has selected projects to develop a pebble bed reactor and a molten chloride fast reactor to receive multi-year cost-share funding worth up to a total of $80 million.” (DOE 2018), and, that “Secretary of Energy Rick Perry Announces $60 Million for U.S. Industry Awards in Support of Advanced Nuclear Technology Development”, which support is to be split between 13 projects in 10 different states (Perry 2018). Although much of this work will be performed in the government’s laboratories by its contractors’ employees (it’s illegal to do genuinely “hot” research elsewhere in the US), it won’t take responsibility for guiding it or establish appropriate goals. Finally, although these projects have accomplished a good deal of computerized modeling, simulation, and “road mapping” (see DOE’s http://gain.inl.gov website), DOE’s nuclear scientists/engineers can’t effectively address the future’s energy issues until they are empowered to do the same sorts of risky/messy experimentation performed during the 1950’s -60s at its “National Reactor Testing Station” (NRTS, now INL). Unfortunately, although those efforts successfully designed, built, operated, and then safely decommissioned ~50 different nuclear reactors, the USA never built a test reactor capable of emulating/ evaluating today’s front-running MSR concepts & is apparently still not planning to do so.Of the approximately 400 papers and posters presented at the IAEA’s latest international conference having to do with “Fast Reactors and Related Fuel Cycles: Next Generation Nuclear Systems for Sustainable Development (FR17)” (IAEA 2018), four presentations were made by people representing the USA – one was about DOE’s road mapping, two about its long defunct LMFBR program, and one by an antinuke anxious to remind everyone that nuclear stuff could be dangerous. One reason for the USA’s poor showing at such meetings is that its regulations criminalize many of the activities intrinsic to international cooperation. Bill Gates recently summed up the situation as follows, “Unfortunately, America is no longer the global leader on nuclear energy that it was 50 years ago. To regain this position, it will need to commit new funding, update regulations, and show investors that it’s serious”(Gates 2018).In contrast to its current commitment to nuclear energy R&D, the USA’s DOE/NNSA spends ~$6.9 billion/a to maintaining its stockpile of nuclear weapons and its total (DOD+DOE) nuclear weapons-related expenditures are on the order of $20–40 billion/a (Rumbaugh and Cohn 2012).The good news is that a large number of relatively (to me anyway) young people (Ecomodernism 2018 & the Weinberg http://Foundation.org ), several environmental groups, and some especially distinguished senior scientists have taken up Goeller and Weinberg’s cause (Pro nuclear 2018).While the West’s “nuclear power resurgence” has slowed to a snail’s pace, China is steadily firing up state-of-the-art light water reactors, its new fast reactor went to full power before Christmas (2014), it has begun construction of a new high-temperature gas-cooled nuclear reactor (Chinese HTR) in April (2015) (Forbes 2015). Most importantly, it has also apparently decided to commit 22 billion yuan ($3.3 billion) to developing the technology abandoned by the US 47 years ago, Weinberg and Wigner’s molten salt reactors (SCMP 2017).China is not leading this particular campaign because it has more experience with nuclear power or can operate reactors more efficiently and safely than do the Western world’s countries. It’s leading because its political leadership has both the will and foresight required to pursue activities apt to serve their county’s best interests over the long haul.. China’s leaders accept the fact that it’s their responsibility to provide a safe, comfortable, and clean world for their descendants and also choose to believe in scientific consensus and that both intra and international cooperation is beneficial. Consequently since circa 1978 they have been demonstrating that it is possible to incentivize its entrepreneurs to do the things required to address China’s (and the world’s) “technical” problems without ceding control/choice of those goals. It’s been done by lending state support to targeted industries and technologies, particularly those infrastructure-related activities required to address issues like those serving as the subject of this essay. We should also remember that China is already heavily involved in African development as a part of its 65-nation “Belt and Road” initiative. The opinion of some Western world politicians that such activity is “greedy and evil” is wrong-headed. It is a fine example of help-your-neighbor globalization, not colonization, and African agency, not Chinese rapacity (Bräutigam 2018).ConclusionsWhile this exercise’s rosy scenario is very likely possible, I don’t consider it realistic because it assumes that quantitative technical arguments, not “human nature”, will determine what actually happens. Collectively, homo sapiens “thinks” more like the yeast cells within a brewing tun than do individual humans like James Hansen. I also didn’t try to address “proliferation”, Chernobyl, Fukushima, the consequences of the USA’s radioactive waste management boondoggling, etc., etc. because they are all manifestations of untrammeled human nature, not “technical” issuesReferencesAgecon. 2015, https://agecon.unl.edu/cornhusker-economics/2015/fuel-prices-and-cost-of-production.Agrawal, R., Singh, N. R., Ribeiro, F. H., and Delgass, W. N. 2007. “Sustainable fuel for the transportation sector”, PNAS, March 20, vol. 104, no. 12, 4828–4833 doi 10.1073 pnas.0609921104.Alvarez, R. A., Pacala, S. W., Winebrake, J. J., Chameides, W. L., and Hamburg, S. P. 2012.” Greater focus needed on methane leakage from natural gas infrastructure”, PNAS, April 24, 2012. 109 (17) 6435-6440; Greater focus needed on methane leakage from natural gas infrastructure).Barriers to Science. 1994, “ Technical Management of the Department of Energy Environmental Remediation Program” , NAP Press The National Academies PressBeerling, D. J., Leake, J. R., Long, S. P., Scholes, J. D., Ton J. Nelson, P.N., Bird, M., Kantzas, E., Taylor L. L., Sarkar, B., Kelland, M., DeLucia, E., Kantola, I., Müller, C., Rau, G. H., and James Hansen. 2018. “Farming with crops and rocks to address global climate, food and soil security”, Nature Plants, volume 4, pages138–147 ( also see http://lc3m.org/news/. )Bettis, E. S., and Robertson R. C. 1970. “The Design and Performance Features of a Single Fluid Molten Salt Breeder Reactor”, Nuclear Applications and Technology, 8, 2 (February). (Paywalled - free at http://energyfromthorium.com/pdf/NAT_MSBRdesign.pdf).BP 2017, https://knoema.com/smsfgud/bp-world-reserves-of-fossil-fuelsBP. 2018, https://www.bp.com/content/dam/bp/en/corporate/pdf/energy-economics/statistical-review/bp-stats-review-2018-full-report.pdfBräutigam, D. 2018. U.S. politicians get China in Africa all wrong, https://www.washingtonpost.com/news/theworldpost/wp/2018/04/12/china-africa/?noredirect=on&utm_term=.fe641fe6f38fBrook, B.W., Blees T., Wigley, M. L. and Sanghyun Hong, “Silver Buckshot or Bullet: Is a Future ‘Energy Mix’ Necessary?” Sustainability 10(2):302 • January 2018, DOI: 10.3390/su10020302, https://www.researchgate.net/publication/322694071_Silver_Buckshot_or_Bullet_Is_a_Future_Energy_Mix_Necessary.Buffet, W., 2014, https://www.usnews.com/opinion/blogs/nancy-pfotenhauer/2014/05/12/even-warren-buffet-admits-wind-energy-is-a-bad-investmentCampbell, N. S. 2009. PhD thesis University of Glasgow, The use of rockdust and composted materials as soil fertility amendments. http://theses.gla.ac.uk/617/.Cataluña, R., Kuamoto, P. M., Petzhold, C. L., Caramão, E. B., Machado M. E., and da Silva R. 2013. Using Bio-oil Produced by Biomass Pyrolysis as Diesel Fuel, Energy Fuels, 2013, 27 (11), pp 6831–6838, DOI: 10.1021/ef401644v.CATO. 2018. https://www.cato.org/blog/prosperity-world-population-growth.Cheap. 2017. The Cheapest Places to Live in the World - 2018.Clack, T.M, Qvist, S.A., Apt, J., Basilian, M., Brandt, A. R., Caldeira K., et al, “Evaluation of a proposal for reliable low-cost grid power with 100% wind, water, and solar”, Evaluation of a proposal for reliable low-cost grid power with 100% wind, water, and solar.Clark, M., and Tilman, D. 2017. “Comparative analysis of environmental impacts of agricultural production systems, agricultural input efficiency, and food choice”, Environmental Research Letters, Volume 12, Number 6, published 16 June 2017, http://iopscience.iop.org/article/10.1088/1748-9326/aa6cd5/metaCNA 2014, Military Advisory Board, “National Security and the Accelerating Risks of Climate Change”, May 2014, http://www.cna.org/sites/default/files/MAB_2014.pdf.Cohen, B., L., 1983 “Breeder Reactors: A Renewable Energy Source”, Am. J. Phys., 51, 75 (1983)Conly, S., 2015, “Here’s why China’s one-child policy was a good thing”, https://www.bostonglobe.com/opinion/2015/10/31/here-why-china-one-child-policy-was-good-thing/GY4XiQLeYfAZ8e8Y7yFycI/story.html (see also Sarah Conly, “One Child: Do We Have a Right to More?”, (book) Oxford University Press, 2015).CCS 2018 (accessed) https://en.wikipedia.org/wiki/Carbon_capture_and_storageDasgupta, P. 2003. “World Poverty: Causes and Pathways”, Plenary Lecture delivered at the World Bank’s Annual Bank Conference on Development Economics (ABCDE), Bangalore, 21-22 May 2003. Subsequently published in B. Pleskovic and N.H. Stern, eds., World Bank Conference on Development Economics, 2003 (Washington, DC:World Bank), 2004. http://www.econ.cam.ac.uk/people-files/emeritus/pd10000/publications/worldpov.pdfDeffeyes, Kenneth S. & MacGregor, Ian D. (January 1980). "World Uranium Resources". Scientific American. p. 66Demographics 2018 (accessed) https://en.wikipedia.org/wiki/Demographics_of_the_Middle_East.Desalination 2018 (accessed) Desalination - Wikipedia.DOE. 2018. http://www.world-nuclear-news.org/NN-US-invests-in-advanced-reactor-development-1801168.html.Ecomodernism 2018 The Breakthrough InstituteEFN 2018, (Environmentalists for Nuclear Energy) Frame PageEnergy in Africa, 2018, Energy in Africa - WikipediaESA. 2015. https://esa.un.org/unpd/wpp/publications/files/key_findings_wpp_2015.pdf )Extension. 2002. https://www.extension.iastate.edu/sites/www.extension.iastate.edu/files/allamakee/stovervalue.pdf).Fiorina, Carlo 2013 The Molten Salt Fast Reactor as a Fast-Spectrum. Candidate for Thorium Implementation. PhD thesis, University of Milan https://www.politesi.polimi.it/bitstream/10589/74324/1/2013_03_PhD_Fiorina.pdfForbes. 2015. https://www.forbes.com/sites/jamesconca/2015/02/16/can-smrs-lead-the-u-s-into-a-clean-energy-future/#3c70603b31c7.Foreignpolicy. 2013. ( http://www.foreignpolicy.com/articles/2010/08/05/the_ministry_of_oil_defenseFortress. 2011. https://fortress.wa.gov/ecy/publications/documents/1107017.pdf.Gates 2018 What I learned at work this yearGiampietro, M. 2002. Energy use in agriculture, Encyclopedia of Life Sciences, MacMillan Publishers, Nature Publishing Group, 15 pages. www.els.netFrattini, D., Cinti, G., Bidini, G., Desideri, U., Cioffi, R., Jannelli, E. 2016. Renewable Energy 99 472-482).GINI 2018 Central Intelligence AgencyGoeller, H. E and Weinberg, A. M. 1976. The Age of Substitutability, Science, 191, 4228: 683, Feb 20, 1976. (paywalled, original (1974) version available gratis at http://www.osti.gov/scitech/servlets/purl/5045860).Hansen, J., Sato, M., Kharecha, P., Beerling, D., Berner, R., Masson-Delmotte, V., Pagani, M., Raymo, M., Royer, D. L., Zachos, J. C. 2008. Target Atmospheric CO2: Where Should Humanity Aim?, The Open Atmospheric Science Journal. 2: 217–231. arXiv:0804.1126 . Bibcode:2008OASJ....2..217H. doi:10.2174/1874282300802010217.Hansen et al, 2016, “Ice melt, sea level rise and superstorms: evidence from paleoclimate data, climate modeling, and modern observations that 2°C globalwarming could be dangerous”, Atmos. Chem. Phys., 16, 3761–3812, 2016, www.atmos-chem-phys.net/16/3761/2016/ doi:10.5194/acp-16-3761-2016Hartmann, J., West, A. J., Renforth, P., Köhler, P., De La Rocha, C. L. et al. 2013. “Enhanced chemical weathering as a geoengineering strategy to reduce atmospheric carbon, supply nutrients, and mitigate ocean acidification”, Reviews of Geophysics, 51, https://www.researchgate.net/publication/260407810.Holcomb D.E., Flanagan G. F., Patton B. W., Gehin J. C., Howard R. L., and Harrison, T. J., 2011, Fast Spectrum Molten Salt Reactor Options, ORNL TM-201111/105 https://info.ornl.gov/sites/publications/files/Pub29596.pdfHong, Li-Ciao. 2016. “Super El Niño”, ISBN 978-981-10-0527-5 https://www.springer.com/us/book/978981100526)Höök, M., Tang, X. (2013), "Depletion of fossil fuels and anthropogenic climate change: a review", Energy Policy, 52: 797-809Human Power 2018 Human power - WikipediaHussain, A. and Abolaban, F. 2014. Nuclear Desalination: A Viable Option for Producing Fresh Water- Feasibility and Techno-Economic Studies, Life Sci .J ;11(1):301-307 -ISSN:1097-8135)IAEA 2018, “Fast Reactors and Related Fuel Cycles: Next Generation Nuclear Systems for Sustainable Development (FR17)” , Yekaterinburg, Russian Federation, 26-29 June 2017, STI/PUB/1836 (ISBN: 978-92-0-108618-1)260 pages Fast Reactors and Related Fuel Cycles: Next Generation Nuclear Systems for Sustainable Development (FR17)Israel. 2018. (accessed) https://en.wikipedia.org/wiki/Water_supply_and_sanitation_in_IsraelJackson, R.B., C Le Quéré C., Andrew, R., M., Canadell, J. G., Korsbakken, J. I., Liu, Z, Peters , G P and B Zheng, B., Global Energy Growth Is Outpacing Decarbonization. Environmental Research Letters, 2018 DOI: 10.1088/1748-9326/af303Jacobson, M. Z. and Delucchi, M. A. 2009. A plan to power 100 percent of the planet with renewables, Scientific American, Nov. issue.Jacobson, M. Z., Delucchi, M. A., Zack, A.F. Bauer, Z. A. F., Goodman, S. C.,. Chapman, W. E. et al. 2017. “100% Clean and renewable wind, water, and sunlight (WWS) all sector energy roadmaps for 139 countries of the world,”, January 27, 2017 , http://web.stanford.edu/group/efmh/jacobson/Articles/I/CountriesWWS.pdf.Jankovic, A. 2003. “Variables affecting the fine grinding of minerals using stirred mills”, Minerals Engineering, Vol 16, Issue 4, April 2003, 337-345Jewish, 2016. http://www.jewishvirtuallibrary.org/jsource/brief/Water.htmlKanga, D. W., and Holbrook, 2015, J. H., Use of NH3 fuel to achieve deep greenhouse gas reductions from US transportation, Energy Reports, Volume 1, November 2015, Pages 164-168. https://doi.org/10.1016/j.egyr.2015.08.001Kelley, C. P., Mohtadi, S., Cane, M. A., Seager, R., & Kushnir, Y., “Climate change in the Fertile Crescent and implications of the recent Syrian drough”t, PNAS March 17, 2015 112 (11) http://3241-3246www.pnas.org/cgi/doi/10.1073/pnas.1421533112Kharecha, P.A., and J.E. Hansen, 2013: Prevented Mortality and Greenhouse Gas Emissions from Historical and Projected Nuclear Power, Environ. Sci. Technol., 2013, 47 (9), pp 4889–4895, DOI: 10.1021/es3051197Kim, J., Tsouris, C., Oyola, Y., Janke, C. J., Mayes, R. T., Dai, S., et al., 2014, Uptake of uranium from seawater by amidoxime-based polymeric adsorbent: Field experiments, modeling, and updated economic assessment. Industrial and Engineering Chemistry Research, 53(14), 6076-6083. Uptake of Uranium from Seawater by Amidoxime-Based Polymeric Adsorbent: Field Experiments, Modeling, and Updated Economic AssessmentLal, R. 2008. Sequestration of atmospheric CO2 in global carbon pools, Energy & Environmental Science, July issue, DOI: 10.1039/B809492FLal, R. 2017. Improving Soil Health and Human Protein Nutrition by Pulses-Based Cropping Systems, Advances in Agronomy (Article in press), Advances in Agronomy, 2017 Elsevier Inc., ISSN 0065-2113, http://dx.doi.org/10.1016/bs.agron.2017.05.003Lallanilla, M., 2018 “Facts About Fracking”, Facts About FrackingLeeman, W. P. 1982. “Olivine tholeiitic basalts of the Snake River Plain, Idaho”, in B. Bonnichsen and R. M. Breckenridge, Eds., Cenozoic Geology of Idaho, Idaho Bureau of Mines and Geology Bulletin 26, pp. 181–191 (also characterizes other US basalts), http://geology.isu.edu/Digital_Geology_Idaho/papers/B-26Ch4-1.LLNL, 2015, Lawrence Livermore National Laboratory’s annual energy flow charts (Sanky-diagrams, see https://flowcharts.llnl.gov/) are based upon DOE/EIA figures which consider both data and assumptions regarding the efficiency with which relevant “sectors” (residential, commercial, industrial, and transportation) convert primary energy to useful “energy services” useful. Recent assumptions have varied more than the data.Lovering, J. R., Yip, A. & Nordhaus, T., 2016, Historical construction costs of global nuclear power reactors. Energy Policy, 91, 371–382Mahaffey, J. 2014. “Atomic Accidents”, Chapter 6, Pegasus Books, 442 p.Martin, R. 2015. “Terrapower quietly explores new nuclear reactor strategy”, MIT Technology Review. Retrieved 2015-11-30.Martin, R., 2016, “The dubious promise of bioenergy plus carbon capture”,MITTechnology Review,Bioenergy Plus Carbon Capture Sounds Promising, Looks DubiousMcArthur 2013. Good Things Grow in Scaled Packages: Africa’s Agricultural Challenge in Historical ContextMoore III, B., and B. H. Braswell 1994, “The lifetime of excess atmospheric carbon dioxide”, Global Biogeochem. Cycles, 8(1), 23–38, doi:10.1029/93GB03392Moulton, K. L.; West, J.; Berner, R. A., 2000, “Solute flux and mineral mass balance approaches to the quantification of plant effects on silicate weathering”. Am. J. Sci. 2000, 300 (7), 539−570.Moltex 2018, http://www.moltexenergy.com/learnmore/An_Introduction_Moltex_Energy_Technology_Portfolio.pdf.MSRE 2018. https://en.wikipedia.org/wiki/Molten-Salt_Reactor_ExperimentNCA4 2018, “Fourth National Climate Assessment”, see both https://nca2018.globalchange.gov/ andhttps://science2017.globalchange.gov/Ojuso, J. O. 1990. “Data Bank: the iso-radiation map of Nigeria”, Solar and wind technology, 7:563-575)OMB 2017, https://www.whitehouse.gov/sites/whitehouse.gov/files/omb/sequestration_reports/FY_2018_Sequestration_Update_8-18-17.pdfPerry. 2018. https://www.energy.gov/articles/secretary-energy-rick-perry-announces-60-million-us-industry-awards-support-advancedPimentel, D., Marklein, A., Toth, M. A, Karpoff, M. N., Oaul, G. S., McCormick R, Kyriazis ,J., and Krueger T. 2009. “Food versus fuels –environmental and economic costs”, DOI 10.1007/s10745-009-9215-8 http://www.stopogm.net/sites/stopogm.net/files/foodvsbiofuelspimentel.pdfPresalt 2018 Pre-salt layer - Wikipedia.Priyono, J. and Gilkes R. J. 2004. “Dissolution of milled-silicate rock fertilizers in the soil”, Australian Journal of Soil Research 42(4) · January 2004 DOI: 10.1071/SR03138Pro nuclear 2018 Pro-nuclear movement - WikipediaPSU. 2018. (accessed) Nitrogen Fertilization of CornRawls, J. 2010. “Implications for Waste Handling of the Multiplier Module”, June 29, 2010 http://www.nwtrb.gov/meetings/2010/june/rawls.pdfRedbook 2014. http://www.oecd-nea.org/ndd/pubs/2014/7209-uranium-2014.pdf.)Regalbuto, c., 2014, “Past and Future Efforts to Extract Uranium from Seawater”, March 21, 2014 http://large.stanford.edu/courses/2014/ph241/regalbuto2/RO 2018 (accessed) https://en.wikipedia.org/wiki/Reverse_osmosisRumbaugh, R, and Cohn, N., 2012, “Resolving Ambiguity: Costing Nuclear Weapons,” Stimson Center, May 2012, http://www.stimson.org/images/uploads/Resolving_Ambiguity.pdfSchumacher, E. F., 1973, “Small Is Beautiful: A Study of Economics As If People Mattered”, HarperCollins, HB171.S384 1989SCMP 2017 . http://www.scmp.com/news/china/society/article/2122977/china-hopes-cold-war-nuclear-energy-tech-will-power-warshipsScott, Ian, 2014, UK Patent application GB 2508537 A, https://patentimages.storage.googleapis.com/pdfs/65e7697f591b41905b81/GB2508537A.pdf ) .Siddiqui F., 2018, “No, the Private Sector Won’t Pick Up the Slack for Federal Investments in Energy Innovation”, https://medium.com/third-way/no-the-private-sector-wont-pick-up-the-slack- for-federal-investments-in-energy-innovation-4b3c2029670aSiemer, D., 2015, “Why the MSFR is the “Best” GEN IV Reactor”, Energy Science and Engineering, Volume 3, Issue 2, Feb 2015, pp 83-97. http://onlinelibrary.wiley.com/doi/10.1002/ese3.59/fullSiemer, D., 2019, “Silicate Weathering to Mitigate Climate Change”, in Soil and Climate, Eds. R. Lal and B.A. Stewart, Taylor and Francis, pp 249-265.Sims, R. E. H. 2011. ENERGY-SMART FOOD FOR PEOPLE ANDCLIMATE, FAO Issue Paper http://www.fao.org/docrep/014/i2454e/i2454e00.pdfSmil, V. 2008. “Energy in nature and society- general energetic of complex systems”, (book) MIT Press, Cambridge, MA. 512 pp.Southern Company and TerraPower Prep for Testing on Molten Salt Reactor, DOE Office of Nuclear Energy press release, 9 Aug. 2018 Southern Company and TerraPower Prep for Testing on Molten Salt ReactorSpringer, N., Duchin, F., 2014, “Feeding nine billion people sustainably: conserving land and water through shifting diets and changes in technologies”, Environ. Sci. Technol., 48, 4444−4451, Feeding Nine Billion People Sustainably: Conserving Land and Water through Shifting Diets and Changes in TechnologiesStatista. 2017. Global grain production, 2017 | StatisticSteffen,W. 2018. (coauthors: Johan Rockström, Katherine Richardson, Timothy M. Lenton, Carl Folke, Diana Liverman, Colin P. Summerhayes, Anthony D. Barnosky, Sarah E. Cornell, Michel Crucifix, Jonathan F. Donges, Ingo Fetzer, Steven J. Lade, Marten Scheffer, Ricarda Winkelmann, and Hans Joachim Schellnhuber), Trajectories of the Earth System in the Anthropocene. PNAS, 2018 DOI: 10.1073/pnas.1810141115Stern, R.J., “United States cost of military force projection in the Persian Gulf, 1976–200”. Energy Policy, (2010), doi:10.1016/j.enpol.2010.01.013Tamada, M., 2009, (slides) Collection of uranium from seawater, 2009.11.5TM / IAEA ViennaTamada, M., 2009 (seminar) “Current status of technology for collection of uranium from seawater” http://ecolo.org/documents/documents_in_english/uranium-sea-09_Tamada.pdfTaylor, L.L., Beerling, D. J., Quegan, S., and. Banwart, S. A.,2017, “Simulating carbon capture by enhanced weathering with croplands: an overview of key processes highlighting areas of future model development”, Biol Lettv.13(4); 2017 AprPMC5414688 https://www.ncbi.nlm.nih.gov/pmc/articles/PMC5414688/TECDOC 1647, 2010, “Progress in radioactive graphite waste management”. IAEA , 2010 http://www-pub.iaea.org/MTCD/tcTomlinson, J., 2018, “Nigerian briefing: how engineers can help secure a sustainable economy”, Proceedings of the Institution of Civil Engineers – Energy, https://doi.org/10.1680/jener.17.00024Trump 2018, World Infuriated by Trump's 'Shithole Countries' RemarkUlmer-Scholle, D., S., 2018, “Uranium — Where Is It Found?” Uranium: Where Is It?UNDP. 2018. http://hdr.undp.org/en/content/human-development-index-hdiVan Huis, A. 2012. “Potential of Insects as Food and Feed in Assuring Food Security”, Annual Review of Entomology, 563-582, DOI: 10.1146Weinberg, Alvin (1994). “The First Nuclear Era: The Life and Times of a Technological Fixer”. New York: AIP Press. ISBN 1-56396-358-Wood. 2018. Wood economy - WikipediaWorld Nuclear. 2018. http://www.world-nuclear.org/information-library/nuclear-fuel-cycle/nuclear-power-reactors/generation-iv-nuclear-reactors.aspxZapata, F., and Roy, R. N. (Eds.), 2004, « The use of phosphate rocks for sustainable agriculture », (a joint publication of the AO Land and Water Développement Division and the International Atomic Energy Agency) Food and Agriculture Organisation of the United Nations, Rome Use of phosphate rocks for sustainable agriculture
- Home >
- Catalog >
- Education >
- Chemistry Chart >
- Electronegativity Chart >
- The Periodic Table Of The Elements With Electronegativities >
- Be Able To Describe Periodic Trends Why Do Ions