The Guide of editing Unit 4 Text Questions Online
If you are curious about Tailorize and create a Unit 4 Text Questions, here are the simple ways you need to follow:
- Hit the "Get Form" Button on this page.
- Wait in a petient way for the upload of your Unit 4 Text Questions.
- You can erase, text, sign or highlight of your choice.
- Click "Download" to preserver the documents.
A Revolutionary Tool to Edit and Create Unit 4 Text Questions
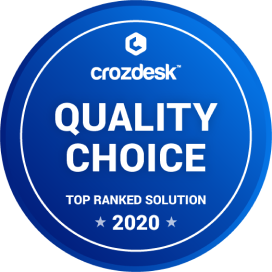
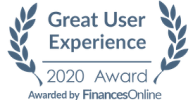
How to Easily Edit Unit 4 Text Questions Online
CocoDoc has made it easier for people to Customize their important documents via online website. They can easily Alter through their choices. To know the process of editing PDF document or application across the online platform, you need to follow these simple ways:
- Open the official website of CocoDoc on their device's browser.
- Hit "Edit PDF Online" button and Import the PDF file from the device without even logging in through an account.
- Edit your PDF document online by using this toolbar.
- Once done, they can save the document from the platform.
Once the document is edited using online website, the user can easily export the document according to your ideas. CocoDoc ensures the high-security and smooth environment for implementing the PDF documents.
How to Edit and Download Unit 4 Text Questions on Windows
Windows users are very common throughout the world. They have met lots of applications that have offered them services in managing PDF documents. However, they have always missed an important feature within these applications. CocoDoc are willing to offer Windows users the ultimate experience of editing their documents across their online interface.
The steps of editing a PDF document with CocoDoc is very simple. You need to follow these steps.
- Choose and Install CocoDoc from your Windows Store.
- Open the software to Select the PDF file from your Windows device and go ahead editing the document.
- Customize the PDF file with the appropriate toolkit presented at CocoDoc.
- Over completion, Hit "Download" to conserve the changes.
A Guide of Editing Unit 4 Text Questions on Mac
CocoDoc has brought an impressive solution for people who own a Mac. It has allowed them to have their documents edited quickly. Mac users can fill PDF form with the help of the online platform provided by CocoDoc.
In order to learn the process of editing form with CocoDoc, you should look across the steps presented as follows:
- Install CocoDoc on you Mac firstly.
- Once the tool is opened, the user can upload their PDF file from the Mac in minutes.
- Drag and Drop the file, or choose file by mouse-clicking "Choose File" button and start editing.
- save the file on your device.
Mac users can export their resulting files in various ways. They can download it across devices, add it to cloud storage and even share it with others via email. They are provided with the opportunity of editting file through various methods without downloading any tool within their device.
A Guide of Editing Unit 4 Text Questions on G Suite
Google Workplace is a powerful platform that has connected officials of a single workplace in a unique manner. If users want to share file across the platform, they are interconnected in covering all major tasks that can be carried out within a physical workplace.
follow the steps to eidt Unit 4 Text Questions on G Suite
- move toward Google Workspace Marketplace and Install CocoDoc add-on.
- Select the file and Press "Open with" in Google Drive.
- Moving forward to edit the document with the CocoDoc present in the PDF editing window.
- When the file is edited completely, download it through the platform.
PDF Editor FAQ
Why is the speed of light a power of 4 (c^4) in general relativity theory?
Because that's what it needs to be for the units to work out.No, really.The [math]c^4[/math] in question is the one on the right hand side of the Einstein Field Equations, which reads:[math]\displaystyle R_{\mu \nu} -\frac{1}{2} g_{\mu \nu} R + g_{\mu \nu} \Lambda = \frac{8\pi G}{c^4} T_{\mu \nu} \tag*{}[/math]Where[math]R_{\mu \nu}[/math] is the Ricci tensor — a function of the second coordinate derivatives and squared first derivatives of [math]g_{\mu \nu}[/math][math]R = R_\sigma^\sigma[/math] is the Ricci scalar — a summation of the above tensor.[math]g_{\mu \nu}[/math] is the metric tensor, the “ruler” of our spacetime.[math]\Lambda [/math] is the cosmological constant (we can ignore this for now)[math]G[/math] is Newton's gravitational constant, [math]6.674\times 10^{-11} [/math]N m[math]^2[/math]/kg[math]^2[/math][math]c[/math] is the speed of light, [math]299792458[/math]m/s by definition[math]T_{\mu \nu}[/math] is the stress-energy tensor(I'm sure you don't need me to define [math]\pi[/math] or [math]8[/math] for you!)So let's check up on our units.[math]g_{\mu \nu}[/math] is dimensionless — it is how we define our rulers, so it cannot be dependent on any units[math]R_{\mu \nu} [/math] is a function of various versions of [math]\frac{\partial^2 g_{\mu \nu}}{\partial x_{\mu}\partial x_{\nu}}[/math] and [math]\left(\frac{\partial g_{\mu \nu}}{\partial x_\mu}\right)^2[/math], and so on. Each copy of [math]\frac{\partial}{\partial x_\sigma}[/math] adds units of one-over-length.Hence, the left hand side of the EFE has units of :[math]\displaystyle LHS = \frac{1}{(\text{length})^2} \tag*{}[/math]For this to be a valid equation, we need the right hand side to have the same units — or else all hell breaks lose.Let's continue:We can see the units of [math]G[/math] from the expression given up there — it's [math]\frac{\text{forces} \times \text{length}^2}{\text{mass}^2}[/math][math]8\pi[/math] is unitless[math]T_{\mu \nu}[/math] has units of energy per cubic metre. This can be seen because [math]T_{00} =\rho[/math], the energy density.Hence, just this combination, with no [math]c^4[/math] term has units:[math]\displaystyle \frac{\text{force} \times \text{energy}}{\text{mass}^2 \times \text{length}} \tag*{}[/math]This looks intractable, but we recall that:The SI base units of energy are: [math]1\text{J} = 1 \text{kg m}^2\text{ s}^{-2}[/math]So [math]\text{energy} = \text{mass}\times \text{speed}^2[/math]Force has units of [math]\text{energy}/\text{distance}[/math],So [math]\text{force} = \text{mass} \times \text{speed}^2 \times \text{length}^{-1}[/math]Therefore if we out the right hand side together, we have:[math]\displaystyle RHS = \frac{\text{force} \times \text{energy}}{\text{mass}^2 \times \text{length}} = \frac{\text{mass}^2 \times \text{speed}^4}{\text{mass}^2\times \text{length}^2} \tag*{}[/math][math]\displaystyle RHS = \text{speed}^4 \times LHS \tag*{}[/math]Hence we see that without the [math]c^4[/math] term, our units are out by a factor of some quantity with units [math]\text{speed}^4[/math]We therefore have a number of choices:This quantity is some physical speed associated with the systemThis quantity is a combination of several fundamental quantities resulting in the units provided.Option 1 is actually ruled out by the derivation of the equation — we've already put everything physical into the other terms.Hence, it can only be some fundamental constants, not physical properties.The only combination of fundamental units that has units of speed-to-the-fourth-power is….[math]\displaystyle c^4\tag*{}[/math]Hence, we need that factor in the equations such that our units on either side of the equals sign are equivalent, which means that the numerical values being equivalent actually has some meaning.
What determines the speed of light? Why is the speed of a massless particle a fixed constant, independent of particle type, and why is this speed so integral to Relativity?
Robert Love claims that, "The speed of light is what it is and it could be nothing else," and that it "It really isn't a speed," which I think is missing out on most of what you can get from this question. There is nothing wrong with calling the speed of light a speed. You can measure the speed of light by putting a mirror far away and timing how long it takes light to bounce off it and come back. If you contort your thinking until that's not a speed, you've confused yourself badly.It is quite useful to work in units where the speed of light is one, but my claim is that Robert's answer interprets this backwards - these are mere units, not physics. Changing units doesn't change physical quantities like speeds. Though you can certainly declare that from now on, a pound is 20 ounces (and therefore your scale reading in pounds goes down), this doesn't mean you lost any weight. Setting [math]c=1[/math] is mere convenience, not a deep insight. This answer will not use units where [math]c=1[/math], and instead use the perfectly-serviceable, if relativistically awkward, meter and second.In regards to four-dimensional spacetime and null geodesics, that is all true. It points out that the speed of light is special because it is invariant between frames. But it doesn't mean that light doesn't have a speed, just that its speed is always the same.Before we get to the physics, there is an important lesson to take away from thinking about units, which is that things in general are measured relative to each other. If I tell you that my friend is tall, you will think he is tall compared to other humans, and is perhaps 2 meters tall. You won't think he is tall compared to skyscrapers. Further, perhaps my friend gets around with other tall guys and says, "boy most people sure are short", which is the same thing as us saying he's tall. So the speed of light is fast compared to most things we encounter, like ping pong balls, jet planes, sloths, etc. We can also say that most things are slow compared to the speed of light. What we want to know is why this is the case.The speed of light is roughly [math]c = 3*10^8\, \text{m/s}[/math], so the speed of light is very fast compared to one meter divided by one second. These are arbitrary measures, but together they form roughly a human walking speed. Your question about the speed of light could be interpreted as asking why light is few hundred million times faster than a walking speed.The speed people walk is overly anthropocentric for physics purposes. Let's choose something more neutral, like the typical speed of sound in a crystal. Light is still fast compared to sound, which is why we see lightning before we hear thunder. (Thunder travels through air, which is not a crystal, of course. Crystals are better for us because the speed of sound in a pure crystal is easy to estimate and does not vary dramatically in everyday circumstances, whereas in a gas the temperature impacts sound speed quite a bit.)The speed of sound in a pure diamond is around [math]10^4\, \text{m/s}[/math]. So the question we'll investigate here is "Why is the speed of light about [math]3*10^4[/math] times faster than the speed of sound in a diamond?"Sound travels through solids as a compression wave. The atoms of the diamond are squeezed together somewhere, adding energy, and this sets up a traveling wave of compressions moving along the crystal from atom to atom. The stiffer the diamond is (more energy to squeeze the atoms), the faster the wave. The more inertia (higher mass per atom), the slower the wave. The only dimensionally-correct way to combine these to get a speed is [math]v = \sqrt{\frac{E}{m}}[/math] where [math]E[/math] is the energy per atom and [math]m[/math] is the mass per atom. (This can be done with more detailed physics, but for this question we're concerned with back-of-the-envelope understanding. This estimate is quite close to the measured value.)We have reduced our problem about understanding why the speed of light is fast to understanding atomic masses and electron binding energies. The mass just comes from the mass of nuclear particles; there is no deep explanation for it, except that that is what the masses and binding energies of quarks turns out to be in quantum chromodynamics. (see Leo and Frank's answers at https://www.quora.com/Particle-Physics/What-is-the-gluon-binding-energy-for-protons-and-neutrons-in-laymans-terms)The energy in an atomic bond comes from quantum mechanics. You can find it by balancing the electrostatic energy between an electron and a proton with the kinetic energy the electron has due to being confined to a region near the nucleus. So the energy depends on the strength of electric interactions, the electron mass, and Planck's constant. Putting them together, you find that the energy is [math]E = \alpha^2 m_e c^2[/math] where [math]\alpha = \frac{e^2}{\hbar c}[/math] is the fine structure constant, [math]m_e[/math] is the mass of the electron, and [math]c[/math] is the speed of light. (see David Simmons-Duffin's answer to What are some examples where solving a problem with just dimensional analysis can make things go wrong?)Putting this together, we find [math]v = c\alpha \sqrt{\frac{m_e}{m_N}}[/math] where [math]m_N[/math] is the mass of a nucleus. Carbon nuclei are some twenty thousand times the mass of an electron and the fine structure constant is around .007, so that expression gives [math]v \approx c\,* 5 * 10^{-5}[/math]. This matches up pretty well with the [math]10^4 \,\text{m/s}[/math] figure, since [math]c\;* 5*10^{-5} \approx 1.5*10^4 \,\text{m/s}[/math]. Our principal cheat was using the ionization energy of hydrogen to describe carbon, and we lucked out a bit to get within a factor of 1.5. Still, to order of magnitude we now have a good understanding of sound speeds in terms of the speed of light.In other words, the speed of light is [math]10^4[/math] or [math]10^5[/math] times faster than the speed of sound in a crystal because the fine structure constant is small and because electrons are light compared to nuclei. There are no good deeper reasons for this that we know of (no anthropic arguments please!). It would be nice to go deeper, but I'm not sure we can at the moment. So that is why the speed of light is so fast, or equivalently why the speed of sound is so slow. It's just because some accidents of the universe happen to have fairly-small numbers that combine to make it so.This might look like an overly-specialized calculation. Why pick this particular thing, the speed of sound, and fixate on it? In truth it is not very special. If you burned some gasoline and measured the thermal velocity of the carbon, it would be roughly the same because the energies involved are the same binding energies of electrons. Except a few nuclear processes and a few gravitational ones, everything around us is ruled by chemistry, so the speeds we see are limited by chemical energies, and these energies are small. So the speeds are slow, or equivalently, the speed of light is fast.adapted from my answer on physics.stackexchange here: http://physics.stackexchange.com/a/57006/74
How bright would the interface region between an antimatter Galaxy and a matter Galaxy be?
This question is a follow up to my answer to How do we know that some galaxies are not made entirely of anti-matter?In that answer, I state that the reason that we know distant galaxies are not made of antimatter is that space is not entirely empty, and hence a “matter galaxy” and an “antimatter galaxy” would have a cloud of matter and antimatter surrounding each of them respectively.If there is a portion (or portions) of our universe made of antimatter, then at some point in space, these two regions must overlap.The interface between these two regions would give off a large amount of gamma radiation, as the two types of matter interacted, and annihilated each other.That we don’t see this gamma-ray-glow is evidence against the theory that distant galaxies are made of antimatter.In the answer, I even quoted some figures — which I aim to explain here, and hence, ultimately, answer the question:Exactly how bright would it be?To calculate the power output, we first need to work out the rate of matter-antimatter collisions.Simple dimensional arguments show us that the formula we need to use to calculate this quantity is as follows:[math]\displaystyle R = n_m n_a v \sigma_{am} \tag*{}[/math]Where:[math]n_m[/math] is the number density (number of particles per [math]m^3[/math]) of matter[math]n_a[/math] is the number density of antimatter[math]v[/math] is the speed of matter as viewed by the antimatter (or vice versa)[math]\sigma_{am}[/math] is the collisional cross section between matter and antimatterThese first two quantities are approximately equal, and are the density of the intergalactic medium (IGM), which is approximately one proton per cubic metre:[math]\displaystyle n_m \approx n_a \approx 1 \text{m}^{-3} \tag*{}[/math][math]v[/math] is the speed of the net motion of the matter and antimatter particles as they collide.We can take this to be simply the velocity of the particles due to their thermal motion. The IGM is surprisingly hot, about [math]10^7[/math]K (there’s nothing to slow particles down, which is why it’s so hot), which means that they have an associated velocity of around:[math]\displaystyle v \approx \sqrt{\frac{k_b T}{m_p}} = 300 \text{km/s} \tag*{}[/math]Now, [math]\sigma[/math] is a harder thing to pin down, it is (roughly) the “size” that the proton has, as viewed by the antiproton. The cross section of a classical ball is just [math]\pi r^2[/math]. But protons are quantum objects, which means the physics is horrible and complicated, and more importantly changes with energy. A proton-antiproton reaction in the LHC has a very different cross section to the cross section we’re dealing with here.I hate collision cross sections, but I managed to stick with it long enough to find this paper, which says in its abstract:The [math]p\bar{p}[/math] total inelastic cross section has been measured in a hydrogen bubble chamber for p momenta from 100 to 550 MeV/c. Below 200 MeV/c the cross section increases rapidly to several barnsWe are a long, long way below 200MeV/c for our momentum, so we know that it is more than “a couple of barns”. A barn is a unit of area equal to [math]10^{-28}[/math]m[math]^2[/math], so named because of the expression “couldn’t hit the side of a barn” (no, really).Hence, looking at the rest of their data, it does not seem unreasonable to set:[math]\displaystyle \sigma \approx 20 \[/math][math]text[/math][math]{ barn} \tag*{}[/math]We can then plug these values into Wolfram|Alpha, and see that we get a value of:[math]\displaystyle R \approx 1.8 \times 10^{-21} \frac{\text{collisions}}{\text{m}^3 \text{seconds}} \tag*{}[/math]Or:[math]\displaystyle R \approx 3 \times 10^{-14} \frac{collisions}{\text{m}^3 \[/math][math]text[/math][math]{ year}} \tag*{}[/math]That means that in a given 1 cubic metre region of space, we would expect 1 reaction to occur within it every 34 trillion years.That’s a long time.It also sounds like things might not be sounding too good for my answer, after all, a reaction that slow would certainly not be detectable by our telescopes.The answer lies in the fact that space is huge.To calculate the total energy being released by this process, we need to know over what volume it is taking place.It does not seem inconceivable to suggest that the “interface region” is approximately the same size as the galaxy itself.Galaxies are (for the most part) on the order of [math]\approx 100[/math]kpc across (300,000 light years), or at least, the ones that are bright enough so we can see them are!Hence, we can approximate that the surface area of our boundary region is[math]\displaystyle A \approx (100 \text{kpc})^2 = 10^4 \text{kpc}^2 = 10^{11} \text{ly}^2 \tag*{}[/math]We then need to work out how deep this interface region is.This bit is going to be pure guesswork, but we can argue that the separation between neighbouring galaxies is going to be at least the size of the galaxies themselves.The region closest to each galaxy is going to be “pure” matter (or antimatter), but the blurring will start at some non-trivial distance from the galaxy.Without any further information, we can just say “the interface region will be on the scale of intergalactic distances”.This scale is, as before, around [math]100[/math]kpc — so we get the volume of our interface region to be:[math]\displaystyle V \approx (100 \text{kpc})^3 = 10^6 \text{kpc}^3 = 3.5 \times 10^{16} \text{ly}^3 \tag*{}[/math]Therefore, the number of annihilation reactions per second in the interface region is simply:[math] \mathcal{R} = R \times V \tag*{}[/math][math]\mathcal{R} = 1.8 \times 10^{-21} \frac{\text{collisions}}{\text{m}^3 \text{seconds}} \times 10^6 (kpc)^3 \tag*{}[/math]Simplifying this (and being careful with our units!) gives us:[math]\mathcal{R} \approx 5.33 \times 10^{43} \frac{\text{annihilations}}{\text{second}} \tag*{}[/math]Bloody hell.That is a lot of reactions going on!We took a reaction that was basically nonexistent in a “comprehend-able” size bit of space, and simply because of the sheer size of a galaxy, we can see that there are trillions upon trillions of these reactions occurring per second!What is the total energy output?The total energy produced in this region of space can be found by multiplying by the energy released per reaction.Since we are considering the total annihilation of a proton with an antiproton, the energy release is simply [math]2 m_p c^2[/math], plus their kinetic energy. However, since we’re strictly in the classical regime ([math]v \ll c[/math]), the rest mass totally dominates, so we can all but ignore the kinetic energy.Hence:[math] \displaystyle P = \mathcal{R} \times 2 m_p c^2 \approx 1.6 \times 10^{34} \[/math][math]text[/math][math]{ watts} \tag*{}[/math](You can see the calculation here)OK, that is a lot of power.I won’t even try to put this into human terms: It is comparable to the energy released in a supernova, and is roughly as bright as a galaxy.Of course, it is spread over a much wider region of space than a supernova, so it will appear as less bright, but it would significantly outshine any other background sources, and would be a noticeable feature strung between two galaxies.In fact, because of the isotropy of the universe, if such boundary regions existed, they would totally drown out the cosmic microwave background!So how bright would this interface be?Approximately 0.2% as bright as an entire galaxy. Easily within the realms of our detectors, especially as we would expect this to be a constant, ever present background.
- Home >
- Catalog >
- Education >
- Chemistry Chart >
- Electronegativity Chart >
- The Periodic Table Of The Elements With Electronegativities >
- Unit 4 Text Questions