The Guide of finalizing Design, Build And Test In A Thermal Fluids Laboratory Course Online
If you are curious about Alter and create a Design, Build And Test In A Thermal Fluids Laboratory Course, here are the simple steps you need to follow:
- Hit the "Get Form" Button on this page.
- Wait in a petient way for the upload of your Design, Build And Test In A Thermal Fluids Laboratory Course.
- You can erase, text, sign or highlight of your choice.
- Click "Download" to conserve the files.
A Revolutionary Tool to Edit and Create Design, Build And Test In A Thermal Fluids Laboratory Course
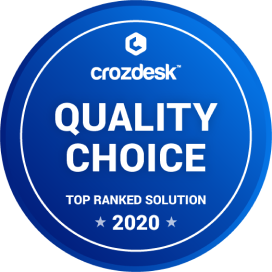
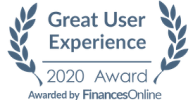
Edit or Convert Your Design, Build And Test In A Thermal Fluids Laboratory Course in Minutes
Get FormHow to Easily Edit Design, Build And Test In A Thermal Fluids Laboratory Course Online
CocoDoc has made it easier for people to Customize their important documents with online website. They can easily Tailorize through their choices. To know the process of editing PDF document or application across the online platform, you need to follow the specified guideline:
- Open the official website of CocoDoc on their device's browser.
- Hit "Edit PDF Online" button and Append the PDF file from the device without even logging in through an account.
- Edit your PDF document online by using this toolbar.
- Once done, they can save the document from the platform.
Once the document is edited using online website, you can download or share the file of your choice. CocoDoc ensures the high-security and smooth environment for implementing the PDF documents.
How to Edit and Download Design, Build And Test In A Thermal Fluids Laboratory Course on Windows
Windows users are very common throughout the world. They have met hundreds of applications that have offered them services in managing PDF documents. However, they have always missed an important feature within these applications. CocoDoc are willing to offer Windows users the ultimate experience of editing their documents across their online interface.
The steps of modifying a PDF document with CocoDoc is very simple. You need to follow these steps.
- Choose and Install CocoDoc from your Windows Store.
- Open the software to Select the PDF file from your Windows device and move on editing the document.
- Customize the PDF file with the appropriate toolkit offered at CocoDoc.
- Over completion, Hit "Download" to conserve the changes.
A Guide of Editing Design, Build And Test In A Thermal Fluids Laboratory Course on Mac
CocoDoc has brought an impressive solution for people who own a Mac. It has allowed them to have their documents edited quickly. Mac users can make a PDF fillable online for free with the help of the online platform provided by CocoDoc.
In order to learn the process of editing form with CocoDoc, you should look across the steps presented as follows:
- Install CocoDoc on you Mac firstly.
- Once the tool is opened, the user can upload their PDF file from the Mac with ease.
- Drag and Drop the file, or choose file by mouse-clicking "Choose File" button and start editing.
- save the file on your device.
Mac users can export their resulting files in various ways. With CocoDoc, not only can it be downloaded and added to cloud storage, but it can also be shared through email.. They are provided with the opportunity of editting file through different ways without downloading any tool within their device.
A Guide of Editing Design, Build And Test In A Thermal Fluids Laboratory Course on G Suite
Google Workplace is a powerful platform that has connected officials of a single workplace in a unique manner. When allowing users to share file across the platform, they are interconnected in covering all major tasks that can be carried out within a physical workplace.
follow the steps to eidt Design, Build And Test In A Thermal Fluids Laboratory Course on G Suite
- move toward Google Workspace Marketplace and Install CocoDoc add-on.
- Select the file and Hit "Open with" in Google Drive.
- Moving forward to edit the document with the CocoDoc present in the PDF editing window.
- When the file is edited completely, download or share it through the platform.
PDF Editor FAQ
Is aerospace engineering a good choice for the future?
Hello; Yes Great choice! Why not?!87.000$ to 114.000$ per year!!!Top 3 aerospace engineer JobsManual Machinist - Roush Industries - Livonia, MIWith over 4,000 employees in facilities throughout the United States, Europe, Asia, and South America, our unique combination of creativity andIT Support Engineer - Roush Industries - Wittmann, AZWith over 4,000 employees in facilities throughout the United States, Europe, Asia, and South America, our unique combination of creativity andPrototype CNC Machinist (Engine Block & Cylinder Head- Roush Industries - Livonia, MIWith over 4,000 employees in facilities throughout the United States, Europe, Asia, and South America, our unique combination of creativity andSee all aerospace engineer jobsWhat Aerospace Engineers Do[About this section]Aerospace engineers design primarily aircraft, spacecraft, satellites, and missiles. In addition, they test prototypes to make sure that they function according to design.Duties of Aerospace EngineersAerospace engineers typically do the following:Direct and coordinate the design, manufacture, and testing of aircraft and aerospace productsAssess proposals for projects to determine if they are technically and financially feasibleDetermine if proposed projects will result in safe aircraft and partsEvaluate designs to see that the products meet engineering principles, customer requirements, and environmental challengesDevelop acceptance criteria for design methods, quality standards, sustainment after delivery, and completion datesEnsure that projects meet quality standardsInspect malfunctioning or damaged products to identify sources of problems and possible solutionsAerospace engineers may develop new technologies for use in aviation, defense systems, and spacecraft. They often specialize in areas such as aerodynamic fluid flow; structural design; guidance, navigation, and control; instrumentation and communication; robotics; and propulsion and combustion.Aerospace engineers can specialize in designing different types of aerospace products, such as commercial and military airplanes and helicopters; remotely piloted aircraft and rotorcraft; spacecraft, including launch vehicles and satellites; and military missiles and rockets.Aerospace engineers often become experts in one or more related fields: aerodynamics, thermodynamics, celestial mechanics, flight mechanics, propulsion, acoustics, and guidance and control systems.Aerospace engineers typically specialize in one of two types of engineering: aeronautical or astronautical.Aeronautical engineers work with aircraft. They are involved primarily in designing aircraft and propulsion systems and in studying the aerodynamic performance of aircraft and construction materials. They work with the theory, technology, and practice of flight within the earth’s atmosphere.Astronautical engineers work with the science and technology of spacecraft and how they perform inside and outside the earth’s atmosphere.Aeronautical and astronautical engineers face different environmental and operational issues in designing aircraft and spacecraft. However, the two fields overlap a great deal because they both depend on the basic principles of physics.Work Environment for Aerospace Engineers[About this section]Aerospace engineers held about 72,500 jobs in 2014. The industries that employed the most aerospace engineers were as follows:They are employed in industries where workers design or build aircraft, missiles, systems for national defense, or spacecraft. Aerospace engineers work primarily for firms that engage in manufacturing, analysis and design, research and development, and for the federal government.Aerospace engineers now spend more of their time in an office environment than they have in the past, because modern aircraft design requires the use of sophisticated computer equipment and software design tools, modeling, and simulations for tests, evaluation, and training.Aerospace engineers work with other professionals involved in designing and building aircraft, spacecraft, and their components. Therefore, they must be able to communicate well, divide work into manageable tasks, and work with others toward a common goal.Aerospace Engineers Work SchedulesAerospace engineers typically work full time. Engineers who direct projects must often work extra hours to monitor progress, to ensure that the design meets requirements, to determine how to measure aircraft performance, to see that production meets design standards, and to ensure that deadlines are met.How to Become an Aerospace Engineer[About this section] [To Top]Get the education you need: Find schools for Aerospace Engineers near you!Aerospace engineers must have a bachelor’s degree in aerospace engineering or another field of engineering or science related to aerospace systems. Aerospace engineers who work on projects that are related to national defense may need a security clearance. U.S. citizenship may be required for certain types and levels of clearances.Aerospace Engineer EducationEntry-level aerospace engineers usually need a bachelor’s degree. High school students interested in studying aerospace engineering should take courses in chemistry, physics, and math, including algebra, trigonometry, and calculus.Bachelor’s degree programs include classroom, laboratory, and field studies in subjects such as general engineering principles, propulsion, stability and control, structures, mechanics, and aerodynamics, which is the study of how air interacts with moving objects.Some colleges and universities offer cooperative programs in partnership with regional businesses, which give students practical experience while they complete their education. Cooperative programs and internships enable students to gain valuable experience and to finance part of their education.At some universities, a student can enroll in a 5-year program that leads to both a bachelor’s degree and a master’s degree upon completion. A graduate degree will allow an engineer to work as an instructor at a university or to do research and development. Programs in aerospace engineering are accredited by ABET.Important Qualities for Aerospace EngineersAnalytical skills. Aerospace engineers must be able to identify design elements that may not meet requirements and then must formulate alternatives to improve the performance of those elements.Business skills. Much of the work done by aerospace engineers involves meeting federal government standards. Meeting these standards often requires knowledge of standard business practices, as well as knowledge of commercial law.Critical-thinking skills. Aerospace engineers must be able to translate a set of issues into requirements and to figure out why a particular design does not work. They must be able to ask the right question, then find an acceptable answer.Math skills. Aerospace engineers use the principles of calculus, trigonometry, and other advanced topics in math for analysis, design, and troubleshooting in their work.Problem-solving skills. Aerospace engineers use their education and experience to upgrade designs and troubleshoot problems when meeting new demands for aircraft, such as increased fuel efficiency or improved safety.Writing skills. Aerospace engineers must be able both to write papers that explain their designs clearly and to create documentation for future reference.Licenses, Certifications, and RegistrationsLicensure is not required for entry-level positions as an aerospace engineer. A Professional Engineering (PE) license, which allows for higher levels of leadership and independence, can be acquired later in one’s career. Licensed engineers are called professional engineers (PEs). A PE can oversee the work of other engineers, sign off on projects, and provide services directly to the public. State licensure generally requires:A degree from an ABET-accredited engineering programA passing score on the Fundamentals of Engineering (FE) examRelevant work experience, typically at least 4 yearsA passing score on the Professional Engineering (PE) examThe initial FE exam can be taken after one earns a bachelor’s degree. Engineers who pass this exam are commonly called engineers in training (EITs) or engineer interns (EIs). After meeting work experience requirements, EITs and EIs can take the second exam, called the Principles and Practice of Engineering.Advancement for Aerospace EngineersEventually, aerospace engineers may advance to become technical specialists or to supervise a team of engineers and technicians. Some may even become engineering managers or move into executive positions, such as program managers.Aerospace Engineer Salaries[About this section] [More salary/earnings info]The median annual wage for aerospace engineers was $105,380 in May 2014. The median wage is the wage at which half the workers in an occupation earned more than that amount and half earned less. The lowest 10 percent earned less than $66,110, and the highest 10 percent earned more than $155,240.In May 2014, the median annual wages for aerospace engineers in the top industries in which they worked were as follows:Aerospace engineers typically work full time. Engineers who direct projects must often work extra hours to monitor progress, to ensure that the design meets requirements, to determine how to measure aircraft performance, to see that production meets design standards, and to ensure that deadlines are met.Job Outlook for Aerospace Engineers[About this section]Employment of aerospace engineers is projected to decline 2 percent from 2014 to 2024. Aircraft are being redesigned to cut down on noise pollution and to raise fuel efficiency, which will help sustain demand for research and development. However, growth will be tempered because many of these engineers are employed in manufacturing industries that are projected to grow slowly or even decline.Most of the work of aerospace engineers involves national defense–related projects or the design of civilian aircraft. Research-and-development projects, such as those related to improving the safety, efficiency, and environmental soundness of aircraft, should sustain demand for workers in this occupation.Aerospace engineers who work on engines or propulsion will continue to be needed as the emphasis in design and production shifts to rebuilding existing aircraft so that they are less noisy and more fuel efficient.In addition, as governments refocus their space efforts, new companies are emerging to provide access to space beyond the access afforded by standard space agencies. The efforts of these companies will include low-orbit and beyond-earth-orbit capabilities for human and robotic space travel. Unmanned aerial vehicles will create some opportunities for aerospace engineers as authorities find domestic uses for them, such as finding missing persons lost in large tracts of forest or helping to put out forest fires.Aerospace Engineers Job ProspectsAerospace engineers who know how to use collaborative engineering tools and processes and who are familiar with modeling, simulation, and robotics should have good opportunities. Employment opportunities also should be favorable for those trained in computational fluid dynamics software, which has enabled companies to test designs in a digital environment, thereby lowering testing costs. Finally, the aging of workers in this occupation should help to create openings in it over the next decade.Employment projections data for Aerospace Engineers, 2014-24Careers Related to Aerospace Engineers[About this section]Aerospace Engineering and Operations TechniciansAerospace engineering and operations technicians operate and maintain equipment used in developing, testing, and producing new aircraft and spacecraft. Increasingly, these workers are using computer-based modeling and simulation tools and processes in their work.Architectural and Engineering ManagersArchitectural and engineering managers plan, direct, and coordinate activities in architectural and engineering companies.Computer Hardware EngineersComputer hardware engineers research, design, develop, and test computer systems and components such as processors, circuit boards, memory devices, networks, and routers. These engineers discover new directions in computer hardware, which generate rapid advances in computer technology.Electrical and Electronics Engineering TechniciansElectrical and electronics engineering technicians help engineers design and develop computers, communications equipment, medical monitoring devices, navigational equipment, and other electrical and electronic equipment. They often work in product evaluation and testing, using measuring and diagnostic devices to adjust, test, and repair equipment. They are also involved in the manufacture and deployment of equipment for automation.Electrical and Electronics EngineersElectrical engineers design, develop, test, and supervise the manufacturing of electrical equipment, such as electric motors, radar and navigation systems, communications systems, and power generation equipment. Electronics engineers design and develop electronic equipment, such as broadcast and communications systems—from portable music players to global positioning systems (GPSs).Industrial EngineersIndustrial engineers find ways to eliminate wastefulness in production processes. They devise efficient systems that integrate workers, machines, materials, information, and energy to make a product or provide a service.Materials EngineersMaterials engineers develop, process, and test materials used to create a wide range of products, from computer chips and aircraft wings to golf clubs and biomedical devices. They study the properties and structures of metals, ceramics, plastics, composites, nanomaterials (extremely small substances), and other substances to create new materials that meet certain mechanical, electrical, and chemical requirements.Mechanical EngineersMechanical engineering is one of the broadest engineering disciplines. Mechanical engineers design, develop, build, and test mechanical and thermal sensors and devices, including tools, engines, and machines.
Is the Saturn V rocket the most precise machine ever built? If not, what is the most precise machine ever built?
I think the far better answer here than Saturn V would be for you to consider a satellite called “Gravity Probe B”.Gravity Probe B (GP-B) was a satellite-based mission which launched on 20 April 2004 on a Delta II rocket. The spaceflight phase lasted until 2005 and was to test generalFrom:Gravity Probe B - Extraordinary TechnologiesTo test Einstein's theory of general relativity, Gravity Probe B must measure two minuscule angles with spinning gyroscopes, floating in space. While the concept of Gravity Probe B is relatively simple, carrying out the experiment required some of the most accurate and sophisticated technology ever developed. In fact, scientists and engineers from Stanford, Lockheed Martin, and NASA had to invent over a dozen totally new technologies in order to meet GP-B’s near-zero constraints, because much of the technology required simply did not exist when the experiment was first suggested in late 1959 - early 1960. Einstein, himself once a patent clerk, would have enjoyed reviewing these extraordinary technologies. This section describes the technologies of the four systems that comprise the heart of the GP-B experiment.World's Most Perfect GyroscopesTo measure the minuscule angles predicted by Einstein’s theory, the GP-B team needed to build a near-perfect gyroscope—one whose spin axis would not drift away from its starting point by more than one hundred-billionth of a degree each hour that it was spinning. By comparison, the spin-axis drift in the most sophisticated Earth-based gyroscopes, found in high-tech aircraft and nuclear submarines, is seven orders of magnitude (more than ten million times) greater than GP-B could allow.Gyro RotorsThree physical characteristics of any gyroscope can cause its spin axis to drift, independently of the general relativity precession predicted by Einstein’s theory:An imbalance in mass or density distribution inside the gyroscopeAn uneven, asymmetrical surface on the outside of the gyroscopeFriction between the bearings and axle of the gyroscope.This meant that a GP-B gyroscope rotor had to be perfectly balanced and homogenous inside, had to be free from any bearings or supports, and had to operate in a vacuum of only a few molecules. After years of work and the invention of new technologies and processes for polishing, measuring sphericity, and coating, the result was a homogenous 1.5-inch sphere of pure fused quartz, polished to within a few atomic layers of perfectly smooth. In fact, the GP-B gyro rotors are now listed in the Guinness Database of World Records as being the roundest objects ever manufactured, topped in sphericity only by neutron stars.The spherical rotors are the heart of each GP-B gyroscope. They were carved out of pure quartz blocks, grown in Brazil and then fused (baked) and refined in a laboratory in Germany. The interior composition of each gyro rotor is homogeneous to within two parts in a million. On its surface, each gyroscope rotor is less than three ten-millionths of an inch from perfect sphericity. This means that every point on the surface of the rotor is the exact same distance from the center of the rotor to within 3x10-7 inches.Here are two ways to imagine how smooth this is. First, compare the GP-B gyro rotor’s smoothness with another smooth object—a compact disk. CDs and DVDs both appear and feel incredibly smooth. The pits on the compact disk's surface, which carry the digital information, are less than 4/100,000ths of an inch deep (one millionth of a meter). However, compared to the GP-B gyroscope, the surface of a CD is like sandpaper. The bumps and valleys on the surface of the GP-B gyroscope are 100 times smaller than those on a CD. Viewed at the same magnification, one could barely see any imperfections on the gyroscope's surface.Alternatively, imagine a GP-B gyroscope enlarged to the size of the Earth. On Earth, the tallest mountains, like Mount Everest, are tens of thousands of feet high. Likewise, the deepest ocean trenches are tens of thousands of feet deep. By contrast, if a GP-B gyroscope were enlarged to the size of the Earth, its tallest mountain or deepest ocean trench would be only eight feet!Finally, a GP-B gyroscope is freed from any mechanical bearings or supports by levitating the spherical rotor within a precisely machined fused-quartz housing cavity. Six electrodes, evenly spaced around the interior of the housing (three in each half), keep the rotor levitated in the housing cavity. During the IOC phase of the mission, a stream of pure helium gas was used to spin up each of the four gyroscopes to approximately 4,000 rpm. After that, all but a few molecules of the helium spin-up gas were evacuated from the housings, and the gyroscopes were left spinning—a mere 32 microns (0.001 inches) from their housing walls, free from any mechanical or fluid supports. During the experiment, the near-perfectly spherical and homogeneous rotors, combined with the highly sophisticated Gyro Suspension System, resulted in an average spin-down time constant of approximately 15,000 years for the four GP-B gyros.The Gyro Suspension System (GSS)The GSS is a marvel of engineering in its own right. To perform its mission successfully, the GSS had to satisfy a number of requirements:Operate over 8 orders of force magnitude. The same system must be able to suspend the gyroscopes on Earth in a 1 g field as well as generate minimal disturbances at the 10-8 g level during data collection. (Note: it takes nearly 1,000 volts to levitate a GP-B gyro rotor here on Earth, whereas in space, it takes less than 100 millivolts—four orders of magnitude less force. This is one of the important reasons why the GP-B experiment had to be carried out in space, rather than in a laboratory on Earth.)Suspend or levitate the gyroscopes reliably. The system must never let a spinning rotor touch the housing. There is sufficient mechanical energy in a rotor spinning at 4,000 rpm to effectively destroy the rotor and housing in such an event.Operate compatibly with the SQUID readout system. The SQUID magnetometers are extremely sensitive. Thus, the suspension system must not interfere with these sensors, both during ground and on orbit operation.Minimize electrostatic torques during science data collection. The suspension system must meet centering requirements with absolutely minimal control effort, and thereby with minimal residual torques on the rotors.Apply controlled torques to the rotor for calibration and initial rotor spin-axis alignment.Act as an accelerometer as part of the “drag-free” translation control system to further minimize classical torques on the rotors by another factor of 106.The GSS was initially designed over the course of two decades of research and development, beginning in the mid 1970s. The initial design was based on a laboratory system that used analog, rather than digital electronics. However, this analog system had a number of issues, including weight, power consumption, adherence to GP-B torque requirements and compatibility with the GPB SQUID readout electronics, that needed to be addressed in order to use this system in a spacecraft. By 1996, with the GP-B launch date then anticipated to be four-six years away, GP-B senior program management made the difficult decision to literally start over from scratch—that is, they decided to design and construct a digital, rather than analog suspension system. A new collaborative GSS team, including engineers and scientists from Stanford and Lockheed Martin was assembled at Stanford, and in record time, the new GSS team designed and built a digital GSS system that met all of the requirements listed above and performed flawlessly throughout the entire GP-B flight mission. Furthermore, the four suspension systems (one for each gyro) have continued to operate perfectly following the depletion of helium from the dewar, since they do not require cryogenic electronics to function.Gyro Readout SystemFor GP-B to “see” the shape and motion of local spacetime accurately, we must be able to monitor the spin axis orientation of the gyroscopes to within 0.5 milliarcseconds, and locate the poles of the gyroscope to within one-billionth of an inch. How can one monitor the spin axis orientation of this near-perfect gyroscope without a physical marker showing where the spin axis is on the gyroscope? The answer lies in a property exhibited by some metals, called “superconductivity.”Superconductivity & The London MomentSuperconductivity was discovered in 1911 by the Dutch physicist H. Kammerlingh Onnes. He found that at temperatures a few degrees above absolute zero, many metals completely lose their electrical resistance. An electric current started in a superconductor ring would flow forever, if the ring were permanently kept cold. But, superconductors also have other interesting properties. In 1948, the theoretical physicist Fritz London predicted that a spinning superconductor would develop a magnetic moment—created by the electrons lagging the lattice of the superconducting metal—which is therefore exactly aligned with its instantaneous spin axis. In 1963, three different groups, including a GP-B graduate student, demonstrated the existence of this London moment experimentally.What is remarkable about this phenomenon (and most fortunate for Gravity Probe B) is that the axis of this magnetic field lines up exactly with the physical axis of the spinning metal. Here was the “marker” Gravity Probe B needed. We coated each quartz gyroscope with a sliver-thin layer of a superconducting metal, called niobium (1,270 nanometers thick). When each niobium-coated gyroscope rotor is spinning, a small magnetic field surrounds it. By monitoring the axis of the magnetic field, Gravity Probe B knows precisely which direction the gyroscope's spin axis is pointing, relative to its housing.Pickup Loops & SQUID MagnetometersThe magnetic field axis is monitored with a special device called a SQUID (Superconducting QUantum Interference Device). The SQUID is connected to a superconducting niobium pickup loop, deposited on the flat surface at the outer edge of one half of the quartz housing in which the gyro rotor spins. Thus, the loop, which senses the gyro’s spin axis orientation, is located on the planar surface where the two halves of the gyro housing are joined. When the gyroscope precesses or tilts, the London moment magnetic field tilts with it, passing through the superconducting loop. This causes a current to flow in the loop—a quantized current. The SQUID detects this change in magnetic field orientation. The SQUID magnetometers are so sensitive that a field change of only one quantum—equivalent to 5 x 10-14 gauss (1/10,000,000,000,000th of the Earth's magnetic field) and corresponding to a gyro tilt of 0.1 milliarcsecond (3x10-8 degrees)—is detectable.Using the London moment to monitor the gyroscope's orientation was the perfect readout scheme for Gravity Probe B—it is extremely sensitive, extremely stable, applicable to a perfect sphere, and-most important, it exerts negligible torques (forces) on the gyroscope.Telescope & Guide StarA 36 centimeter (14 inch) long Cassegrain reflecting astronomical telescope with a focal length of 3.8 meters (12.5 feet), mounted inside the GP-B Probe along the central axis of the spacecraft and dewar, provided the experiment’s required reference to a distant “guide star.” Any change in spin-axis orientation of each of the gyros, as they traveled through the warped and twisted spacetime around the Earth, was measured against this guide star reference to distant spacetime. During the science phase of the mission, the telescope’s job—in conjunction with the Attitude and Translation Control system (ATC)—was to keep the entire spacecraft precisely pointed at the center of the guide star with a pointing deviation in the range of approximately ±200 milliarcseconds (±6x10-5 degrees).Ideally, the telescope should have been aligned with a distant quasar (massive bodies, located in the most distant reaches of the universe, which put out powerful radio emissions), because they appear to be fixed in their position and would thus provide an ideal, stable reference point for measuring gyroscope drift. However, quasars are too dim for any optical telescope this size to track. So, instead, the telescope was focused on a brighter, nearby “guide star.” But, like the Sun, nearby stars move relative to the other stars in our galaxy, and their light diffracts or scatters as it travels through the universe. This situation posed two difficult challenges to the experiment:Creating a means for the telescope to find and remain focused on the exact center of a star whose light is widely diffracted.Choosing a guide star whose motion could be mapped relative to quasars separately, so that the Gravity Probe B gyroscope measurements can ultimately be related to the distant universe.Precision Pointing—Dividing the Guide Star ImageTo satisfy the precision pointing requirements of the GP-B experiment, it was necessary to locate the optical center of the guide star image in the telescope to an accuracy of 0.1 milliarcseconds (3x10-8 degrees). The diffraction limit size of the GP-B telescope is approximately 1.4 arcseconds, which is about 14,000 times larger than the required pointing accuracy, and this presented a formidable challenge to the GP-B team. The solution was to precisely split the image into equal x-axis and y-axis components, and then to divide each of the axis components into two half images whose brightness values could be compared.GP-B accomplished this task by focusing the mirror-reflected starlight onto an Image Divider Assembly (IDA) at the telescope's front end. In the IDA, the starlight was first passed through a beam-splitter (a half-silvered mirror), that forms two separate images, one for the horizontal (x) axis and one for the vertical (y) axis. The half beam from each axis was then focused onto a roof-prism (a prism shaped like a peaked rooftop). Each roof prism sliced its portion of the starlight beam into two half-disks, which were focused onto a pair of silicon photo diodes.The photo diodes converted the light signals from each half-disk to electrical signals which were then compared. If the signals were not equal, the roof-prism was not slicing the image precisely in half. The orientation of the entire spacecraft was then readjusted in real time until the signals were equal and the image was split right down the middle. When this was accomplished for both the x-axis and y-axis halves of the starlight beam, the telescope was locked on the exact center of the guide star. Once tuned up, the Attitude and Translation Control (ATC) system on-board the spacecraft was capable of locking the telescope onto the guide star in less than a minute each time the spacecraft emerged from behind the Earth over the North Pole and the guide star came into view. Once the telescope was locked onto the guide star, the telescope pointing signals were used to compensate for the small pointing deviation to an accuracy of better than 0.1 milliarcseconds (3x10-8 degrees).Choosing a Suitable Guide StarIn order to precisely map the motion of a star relative to a quasar, it was necessary to find a star that met all of the following criteria:Correct position in the heavens for tracking by the on-board telescope (for example, the sun never gets in the way)Shines brightly enough for the on-board telescope to trackIs a sufficiently strong radio source that can be tracked by radio telescopes on EarthIs visually located within a fraction of a degree from the reference quasarIt so happens that stars that are radio sources belong to binary star systems. Because almost half the star systems in the universe are binary, it initially seemed that there would be many good candidates for the guide star. However, out of 1,400 stars that were examined, only three matched all four of the necessary criteria. The star that was chosen as the GP-B guide star is named IM Pegasi (HR 8703).Relating the Guide Star's Motion to a Distant QuasarLike all stars in our galaxy, the position of IM Pegasi as viewed from Earth and our science telescope changes over the course of a year. In fact, IM Pegasi moves around its binary partner in a spiraling pattern, rather than a linear path. The total motion of IM Pegasi in one year alone is 100 times larger than the smallest gyroscope spin axis precession measurable with Gravity Probe B. Thus, throughout the science phase of the mission, the GP-B science telescope was tracking a moving star, but gyros were unaffected by the star's so called “proper motion;” their pointing reference was IM Pegasi's position at the beginning of the experiment. Consequently, in the gyro precession data for each orbit, it is necessary to subtract out the telescope's angle of displacement from its original guide star orientation so that the angular displacements of the gyros can be related to the telescope's initial position, rather than its current position each orbit. For this reason, a very accurate map of the proper motion of IM Pegasi is required in order to complete the GP-B data analysis.Because IM Pegasi is a radio source, its proper motion can be tracked by a sophisticated system of radio telescopes, operating in conjunction with each other. This system is called Very Long Base Interferometry or VLBI. Using radio telescopes from New Mexico to Australia to Germany, acting as a single radio telescope the size of the Earth, a team at the Harvard-Smithsonian Center for Astrophysics (CfA) led by astrophysicist Irwin Shapiro, in collaboration with astrophysicist Norbert Bartel and others from York University in Canada and French astronomer Jean-Francois Lestrade have now mapped the motion of IM Pegasi with respect to a reference quasar over a number of years to an unprecedented level of accuracy. With these measurements the motions of the GP-B gyroscope spin axes can now be related to the reference quasar in the distant universe. However, to ensure the integrity of the GP-B experiment, we added a “blind” component to the GP-B data analysis by requesting that the CfA withhold the proper motion data that will enable us to pinpoint the orbit-by-orbit position of IM Pegasi until the rest of our GP-B data analysis is complete. Therefore, the actual precession angles of the GP-B gyros with respect to the distant universe, will not be known until the end of the data analysis process.Dewar, Porous Plug, and Micro ThrustersOne of the greatest technical challenges for Gravity Probe B was keeping the probe and science instrument precisely at a designated cryogenic temperature, just above absolute zero, of approximately 2.3 kelvin (-270.9 degrees Celsius or -455.5 degrees Fahrenheit) constantly for 16 months or longer. This was accomplished by integrating the probe into a special 2,441 liter (645-gallon) dewar, or thermos, nine feet tall (about the size of a mini van), that is filled with liquid helium. When cooled to nearly absolute zero temperature, liquid helium transforms into a state called “superfluid,” in which it becomes a completely uniform thermal conductor. Only helium exhibits this, and other special properties of superfluidity. The dewar and its payload inside form the main structure around which the GP-B spacecraft was built.The Dewar and liquid helium served two critically important functions in the mission:Maintaining a supercold temperature around the science instrument.Providing a constant stream of gas propellant for precisely controlling the position and attitude of the entire satellite.Maintaining a Cryogenic Experimental EnvironmentIn its 640-kilometer (400-mile) high polar orbit, the GP-B satellite is low enough to be subjected to heat radiating from the Earth's surface, and it is also subjected to alternating hot and cold cycles, as it passes from intense sunlight into the Earth's shadow every ninety-seven minutes. Throughout the life of the mission, key portions of the science instrument had to be maintained at a constant temperature to within five millionths of a degree centigrade.During the mission, the dewar's insulating chamber remained a vacuum, which limited the amount of heat penetrating through the outside wall into the inner chamber containing the science instrument. In addition, it includes several other devices for maintaining the necessary cryogenic temperature:Multilayer insulation—multiple reflective surfaces in the vacuum space to cut down radiationVapor-cooled shields—metal barriers, suitably spaced, cooled by the escaping helium gasSlosh baffles—metal ridges that help suppress tidal motions in the superfluid heliumPorous Plug—invented at Stanford, and engineered for space at NASA Marshall Space Flight Center in Huntsville, AL, Ball Aerospace in Boulder, CO, and the Jet Propulsion Laboratory in Pasadena, CA. This plug allows helium gas to evaporate from the Dewar's inner chamber, while retaining the superfluid liquid helium inside.Using Dewar Helium Boil-off as Thruster PropellantWhen there was still helium in the dewar, virtually no heat could penetrate from the outside wall through the vacuum and multilayer insulation inside. However, a small amount of heat (about as much as is generated by the message indicator lamp on a cell phone) did leak into the Dewar from two sources:Conduction of heat flowing from the top of the Dewar into the liquid heliumRadiation leaking down through the telescope bore into the liquid helium.The porous plug controls the flow of this evaporating helium gas, allowing it to escape from the Dewar, but retaining the superfluid helium inside. The plug is made of a ground-up material resembling pumice. The evaporating helium gas climbed the sides of the inner tank near the plug and collected on its surface, where it evaporated through the pores in the plug, much like sweating in the human body.The evaporating helium provided its own kind of refrigeration. As the helium gas evaporated at the surface of the porous plug, it drew heat out of the liquid helium remaining in the Dewar, thereby balancing the heat flow into the Dewar. You can feel this effect on your skin when you swab your skin with water. As the liquid evaporates off your skin, it draws heat energy with it, leaving your skin a tiny bit cooler than before.The helium gas that escaped through the porous plug was cycled past the shields in the outer layers of the Dewar, cooling them (thus the name, “vapor-cooled shields”), and then it was used as a propellant for eight pairs of micro thrusters that are strategically located at the top and bottom of the spacecraft. Based on data from the on-board telescope and the gyroscope that was used as a proof mass for maintaining a drag-free orbit, the flow of the escaping helium gas was carefully metered through these thrusters in order to precisely control the spacecraft's position. In fact, the position of the entire spacecraft was balanced around the proof mass gyroscope by increasing or decreasing the flow of helium through opposing thrusters, creating a drag-free orbit.
Where can I buy a spaceship?
I believe a spaceship based on rocket science is too expensive for a single person to buy, pilot and maintain but we can explore another type of spaceship which I believe can be much cheaper to design, build, test, pilot and maintain. The kind of spaceship I will be talking about is based on a concept of concentrically counter-rotating electric and magnetic fields. In other words, it is based on electromagnetism.The spaceship in question is saucer-shaped for very good reasons. Electric field is generated by coils shaped like two inverted cones, joined base to base and aluminium plate capacitors imbedded within the structure of an anticlockwise rotating concentric disk. Magnetic field is generated by horseshoe electromagnets mounted at the periphery of the frame of a clockwise rotating saucer-shaped hull. The electric current to the electromagnets is switched on and off at a frequency dependent on the angular velocity of the hull. For the spaceship to take off the ground, the anticlockwise angular velocity of the concentric disk must match the clockwise angular velocity of the hull. This matching of angular velocity takes place at around 580 rpm.Before I show you the image of how the spaceship in question looks like, let me introduce you to another invention that is related to the spaceship in question in terms of mechanical operation. The reason being, this other system has been verified scientistifically by two Russian scientists, namely, Godin and Roschin. They even published their findings on a Russian scientific journal and you can Google it as it is available online. Here it comes.What is its name? It is called Searl's effect generator and below is an image of the version of this generator that was used by Godin and Roschin, together with an excerpt of the paper published by them in regards to their findings.Experimental Research of the MagneticGravity Effects by V. V. Roschin ( [email protected] ) and S. M. Godin ( [email protected] ) Institute for High Temperatures Russian Academy of Science Izhorskaya 13/19 Moscow 127412, Russia [This file is reproduced by courtesy of Alex Frolov.In the present paper the results of the experimental research of Magnetic-Gravity Effects are presented. The abnormal magnetic and thermal changes in the radius of 15 meters from the researched device were measured as well.Introduction: There has been a great interest in examining nonlinear effects in the system of rotating magnetic fields. Such effects have been observed in the device called Searl’s generator or SEG (SEG, Searl Effect Generator) [1-4]. An SEG consists of a series of three rings and rollers that go around those rings. All parts of SEG are based on the Law of the Squares. The rollers revolve around the plates that form the rings, but they do not touch them. There’s a primary north and south pole on the rollers and a primary north and south pole on the plates. Obviously you will have the north pole of the roller attracted to the south pole of the plate.The plate and the rollers have layered structure. The external layer - Titan, then Iron, Nylon and last internal layer was made from Neodymium. John R. R. Searl has supposed that the electrons are given off from the central element (which is Neodymium), and they travel out through other elements. If nylon had not been put there, the SEG would act like a laser and one pulse would go out and it would stop, build up, and another pulse would go out. But, with the nylon being there, nylon acts as a control gate, and that control gate gives you an even flow of electrons throughout the SEG [4]. In [4] it was shown that in the process of magnetization of the plate and rollers, the combination of constant and variable magnetic fields for creating a special wave (sine wave) pattern on a plate surface and rollers surface was used. The basic effects are the rollers selfrunning around a ring plate and reduction of weight up to occurrence of propulsion and flying up of all magnetic system. These effects come about because of a special geometry of experimental setup. It was shown that the work of the device in critical regime is accompanied by biological and real physical phenomena.Experimental Results: The magnetic-gravity converter was built in a laboratory room on three concrete supports at a ground level. The ceiling height the lab room was 3 meters. Besides the presence of the iron-concrete ceiling, in immediate proximity from the magnetic system there was a generator and electric motor, which contained some tens kg of iron and could potentially deform the field’s pattern. The device was started by the electric motor, which accelerated the rotation of the rotor. The revolutions were smoothly increased up to the moment the ammeter included in a circuit of the electric motor started to show zero or lower value of a consumed current or even a presence of the back current. The presence of the back current is detected at approximately 550 rpm. The magnetic moving sensor 14 starts to detect the change in weight of the whole installation at 200 rpm. Afterwards the electric motor is completely disconnected by the electromagnetic muff and the ordinary electrodynamics generator is connected to the basic shaft of the device through the same muff. The rotor of the converter continues to self-accelerate and with the approach to the critical mode of 550 rpm, the weight of the device quickly changes.In addition to the change speed of rotation the weight depend of the power, removed into active load, (the set of ten ordinary electrical water heaters of 1 kW was used) and of the applied polarizing voltage, as well. At the maximum output power equal to 6-7 kW the change of weight G of the whole platform (total weight is about 350 kg), reaches 35 % of the weight in an initial condition G?. A load of more than 7 KW results in a gradual decrease of revolutions and exit from the mode of self-generation with the subsequent complete stop of the rotor. The weight of a platform can be controlled by applying of a high voltage to cellular ring electrodes located at a distance of 10 mm from external surfaces of the rollers. Under the high 20 kV voltage (electrodes negative pole) the increase of taped power in circuit of the basic generator more than 6 KW does not influence G while the revolutions per min is not decreased to 400 rpm. “Tightening” of this effect is observed as well as the effect of hysteresis on G (a kind of “residual induction”).I hope by reading the above excerpt you have seen what happens to Searl's effect generator when in operation. As you can clearly see, the mechanical working of this generator is related to the spaceship in question, especially in terms of counter-rotating fields. Below is the images of the spaceship in question and it was designed, build and tested by Otis T. Carr in 1950s.This is its design and look. As you can clearly see, it saucer shaped. Just like the UFOs that you have been hearing about. Its principles of operating are different from those of rockets and aeroplanes. The interior concentric disk rotate in an anticlockwise direction and generate electric field while in operation. The hull rotates with the electromagnets in a clockwise direction while generating pulses of magnetic field. According to Otis T. Carr, when the angular velocity of the concentric disk match that of the hull, the entire system takes off the ground and can be steered to any direction by varying the strength of the electromagnets when passing past the direction of interest.As you can see, this kind of spaceship is much simpler and cheaper compared to rockets. If thought that is not supported by science then you read the following excerpt from Wikipedia.[267] Froning and Roach (2002) put forward a paper that builds on the work of Puthoff, Haisch and Alcubierre. They used fluid dynamic simulations to model the interaction of a vehicle (like that proposed by Alcubierre) with the zero-point field. Vacuum field perturbations are simulated by fluid field perturbations and the aerodynamic resistance of viscous drag exerted on the interior of the vehicle is compared to the Lorentz force exerted by the zero-point field (a Casimir-like force is exerted on the exterior by unbalanced zero-point radiation pressures). They find that the optimized negative energy required for an Alcubierre drive is where it is a saucer-shaped vehicle with toroidal electromagnetic fields. The EM fields distort the vacuum field perturbations surrounding the craft sufficiently to affect the permeability and permittivity of space.There another invention by Alexy Chekurkov that is worth studying. Below is a video clip about it.A mixed video clip. Alexy's video is also at the end of the video clip below.Let me leave you with the following excerpt from popular mechanics.“Throughout history, many inventions have preceded an understanding of the science that makes them work,” Matthew Hersch, a history of science and technology professor at Harvard University, tells Popular Mechanics. “Engineers often ‘do science’ in the course of their work, just as scientists ‘invent.’ It’s inevitable that we as a species will continue inventing things without a real understanding of how they work, at least until our science catches up.”In my humble opinion, as with Otis T. Carr's invention, science has caught up with it after more than 50 years, since 1959. This is a spaceship that you can even a hire a few electrical engineers and mechanical engineers to design and build it for you. Other technologies that can be used to finish building your spaceship, complete with life support systems are ready and you can get them off the shelf or can be sourced elsewhere. You must start with an elaborate research to fully understand what you are getting into and doing in regards to the technology in question.
- Home >
- Catalog >
- Miscellaneous >
- Checklist Template >
- Baby Shower Checklist >
- baby shower planning guide >
- Design, Build And Test In A Thermal Fluids Laboratory Course