How to Edit The Wsdot Weight Table quickly and easily Online
Start on editing, signing and sharing your Wsdot Weight Table online under the guide of these easy steps:
- Push the Get Form or Get Form Now button on the current page to jump to the PDF editor.
- Wait for a moment before the Wsdot Weight Table is loaded
- Use the tools in the top toolbar to edit the file, and the edits will be saved automatically
- Download your completed file.
The best-rated Tool to Edit and Sign the Wsdot Weight Table
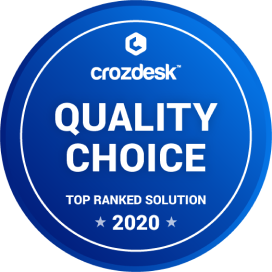
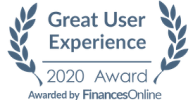
A quick guide on editing Wsdot Weight Table Online
It has become really simple recently to edit your PDF files online, and CocoDoc is the best free PDF editor you would like to use to make a lot of changes to your file and save it. Follow our simple tutorial to start!
- Click the Get Form or Get Form Now button on the current page to start modifying your PDF
- Add, change or delete your text using the editing tools on the toolbar above.
- Affter altering your content, put the date on and add a signature to finalize it.
- Go over it agian your form before you click on the button to download it
How to add a signature on your Wsdot Weight Table
Though most people are adapted to signing paper documents using a pen, electronic signatures are becoming more popular, follow these steps to sign PDF!
- Click the Get Form or Get Form Now button to begin editing on Wsdot Weight Table in CocoDoc PDF editor.
- Click on the Sign tool in the toolbar on the top
- A window will pop up, click Add new signature button and you'll have three ways—Type, Draw, and Upload. Once you're done, click the Save button.
- Drag, resize and settle the signature inside your PDF file
How to add a textbox on your Wsdot Weight Table
If you have the need to add a text box on your PDF and customize your own content, do some easy steps to finish it.
- Open the PDF file in CocoDoc PDF editor.
- Click Text Box on the top toolbar and move your mouse to position it wherever you want to put it.
- Write in the text you need to insert. After you’ve typed the text, you can select it and click on the text editing tools to resize, color or bold the text.
- When you're done, click OK to save it. If you’re not happy with the text, click on the trash can icon to delete it and take up again.
A quick guide to Edit Your Wsdot Weight Table on G Suite
If you are looking about for a solution for PDF editing on G suite, CocoDoc PDF editor is a recommendable tool that can be used directly from Google Drive to create or edit files.
- Find CocoDoc PDF editor and set up the add-on for google drive.
- Right-click on a PDF document in your Google Drive and choose Open With.
- Select CocoDoc PDF on the popup list to open your file with and give CocoDoc access to your google account.
- Modify PDF documents, adding text, images, editing existing text, mark up in highlight, erase, or blackout texts in CocoDoc PDF editor before saving and downloading it.
PDF Editor FAQ
Where can I find sources about dynamic soil compacting?
Washington State Department of TransportationContact WSDOTConcernsLicensing - The Washington State Department of Licensing handles the licensing and regulating drivers, vehicle and boats.Ferries-related 1-888-808-7977 or 206-464-6400Pay a Toll - Good To Go! 1-866-936-8246Report graffitiWebsite feedback and general questionsSpecific areasPrograms and servicesOfficesEmployeesOther inquiriesHow do I?Subscribe/Unsubscribe to emails and text messagesWSDOT Headquarters360-705-7000 / 360-705-7438Staffed 8 a.m.-5 p.m., Monday-Friday except state holidaysMailing Address:Washington State Department of Transportation310 Maple Park Avenue SEP.O. Box 47300Olympia, WA 98504-7300Persons who are hard of hearing may call Washington State Telecommunications Relay Service (TTY) at 711.cost effective method of soil compaction whereby a heavy weight is repeatedly lifted and dropped from a height, impacting the ground surface with a readily calculated impact energy . Costs are reportedly about 2/3 that of stone columns, with up to 50% savings over other deep densification alternatives (Washington State Department of Transportation). Dynamic compaction is one of the oldest soil improvement methods known, reportedly used by the Romans prior to 100 ad and in the United States as early as the 1800s (Welsh, 1986).The name does not accurately portray the actual loading and energy transmission processes. One of the greatest misnomers regarding dynamic compaction is that it is a surface ground treatment, as loads are applied at the surface. But dynamic compaction, as opposed to conventional shallow compaction of controlled fill, is a process of densifying soils at significant depths by applying a large impact energy at the ground surface. Upon impact, craters as deep as six feet or more are created, which must then be backfilled prior to additional compaction passes and ultimately at the completion of the compaction process. But the densification at depth occurs as a result of the dynamic wave energy that is transmitted through the ground.The main objectives of dynamic compaction are to improve strength and compressibility characteristics by either creating a uniform raft of densified material, or by compacting at locations where concentrated loads (e.g., column loads) will be applied. Improved soil properties result in increased bearing capacity and reduced settlements, including differential settlements. Dynamic compaction often allows for construction of conventional spread footings by providing bearing capacity of typically as much as 100-150 kPa (2000-3000 psf).Dynamic compaction is suitable for densification of loose sand deposits such as those typically occurring in coastal, glacial, and alluvial deposits, as well as for dredged or hydraulically placed fills. This method has also been successfully applied to mine tailings, landfills, collapsible soils, sites underlain by sinkholes, and so forth (Zekkos et al., 2013). It is one of the better alternatives to densification of heterogeneous fills, and fills containing large debris that may create obstructions for other remediation techniques, such as stone columns or rigid inclusions (Menard Group, world specialist in ground improvement). Results are best for well-drained, high permeability soils with low saturation, although some satisfactory results have been reported for improvement of silty soils with the aid of PVDs or stone columns (or composite stone columns employing supplemental PVDs), and by providing time delays to allow for the dissipation of generated pore pressures (Dise et al., 1994; Shenthan et al., 2004). In certain conditions, saturated soils will be temporarily liquefied, allowing easier rearrangement and ultimately a tighter, denser packing upon dissipation of pore pressures. Because of this phenomenon and the benefits it can provide, “rest periods” between drop phases are sometimes specified, during which pore pressure dissipation can be monitored with piezometers to assure completion. This method is not appropriate for saturated clay soils.Applications consist of dropping a heavy tamper (weight) from a specified height a calculated number of times at precisely determined locations in a pattern at the site. Drop patterns usually consist of primary and secondary (and occasionally tertiary) grids such as depicted in Figure 6.14. Grid spacing is typically about 3-7 m (9-21 ft). The weights typically range from 6 to 30 tons (up to 40 tons), and the drop heights typically range from 10 to 30 m (30-100 ft), sometimes more.Effective densification is typical to depths of 10 m (or more with very big rigs and weights). The greatest improvement usually occurs between 3 and 8 m (10-25 ft) below the ground surface, with diminishing degrees of improvement at greater depths. The surface layers (surface to approximately 1-3 m) must be recompacted due to the disruption by the impact loads and lack of sufficient confinement. In order to estimate the required compaction effort using dynamic compaction, the Menard formula is generally followed:(6.2)[math]Z=nMH[/math]where Z is the (required) treatment depth, M the tamping mass (tons), H the fall height, n the (soil dependent) constant, typically between 0.3 and 0.6 for sandy soils.Greater depths have been effectively densified using a system known as high energy dynamic compaction, where maximum efficiency is achieved with the complete free fall of the weight through the use of a specially designed weight release system (Menard Group, world specialist in ground improvement). As an extreme case, Menard developed a “Giga” compactor for deeper densification at the Nice airport in France (Figure 6.15).Sign in to download full-size imageFigure 6.15. Menard’s “Giga” compactor drops a 200 ton weight.Courtesy of Menard.Designing a dynamic compaction project application requires determining the most efficient application of energy at the site. This may be initially determined based on data from site investigations. Actual DDC program applications are typically fine-tuned, or modified, based on test sections or after field testing of preliminary applications (i.e., after an initial phase of drops). Field measurements of penetration (or “crater depths”) and pore pressures are continuously monitored to allow for adjustments to the field program. Measurements of crater depths are also used in a manner similar to proof rolling in that deeper crater depths indicate “softer” or “weaker” locations that may require further attention.View chapterPurchase bookSoil compactionAnjan Patel, in Geotechnical Investigations and Improvement of Ground Conditions, 20192.6 Dynamic compactionIn dynamic compaction, a large weight usually of 100–400 kN is dropped repeatedly from a height of about 5–30 m onto the ground using a predetermined grid pattern, as depicted in Fig. 2.6, in order to densify the soil mass or to improve its compactness.Sign in to download full-size imageFig. 2.6. Dynamic compaction. (A) Dropping of weight from a certain weight (Menard), (B) Drop locations.The maximum effective depth of compaction using dynamic compaction can be determined by using the formula as follows:(2.1)[math]Dmax=nWH1/2[/math]where W is the dropped weight in tonnes, H is the height of drop in metres and the value n is a factor that depends upon the type of soil. This depth is also influenced by other factors such as the stratigraphy of the soil, the degree of saturation, the way in which the weight is dropped, and the presence of any damping soil layers. The value of n generally varies from 0.4 to 0.8. The number of drops at each grid point location can be calculated using Eq. (2.2) as follows:(2.2)[math]E=N·W·H·P/S2[/math]where E is the applied energy, N is number of drops at each grid point location, W is dropped weight, H is the height of drop, P is number of passes, and S is the grid spacing. The drop point spacing is generally selected as 1% to 2% times the diameter or width of the dropped block.The dynamic compaction method can be used for various applications such as for the treatment of industrial warehouses, port and airport platforms, roads and railways embankments, heavy storage tanks, nonorganic heterogeneous fill or man-made ground containing larges blocks as obstructions, and soils that are susceptible to liquefaction. The major advantage of adopting the dynamic compaction method is its very high production rate (it can be used to treat an area of > 104m2/month). Moreover, this method is effective both in saturated and nonsaturated soils. In soft ground, the method of dynamic compaction has been proved as a better alternative to preloading, foundation piling, or soil undercutting and replacement.However, the disadvantage or difficulty in adopting the dynamic compaction method is that it requires an intensive in situ testing programme (standard penetration test, cone penetration test, pressure meter test or load settlement test, etc.) to verify that the desired improvement has been achieved in the field. Moreover, constant monitoring is required to check for the formation of crater, ground heave, ground vibration, induced settlement, and pore pressure development during the operation of this method on the site. This method becomes more complicated in silty and clayey deposits below the groundwater due to its relatively low permeability. In such cases, if the energy level is very high, instead of densifying the soil mass, it may bring the ground to a liquefaction state for a longer duration with negligible pore pressure dissipation. To tackle this problem wick drains associated with dynamic compaction are sometimes provided. Also, the dropping of the weight can be scheduled into phases allowing a certain period of time for the dissipation of pore pressures at each drop point location. Otherwise, the dynamic compaction method is modified to what is known as high energy pillars, where, with the help of high tamping energy, large-diameter stone pillars are created in a soft soil. In cases where the water table is close to the ground surface, dewatering is done to lower the level temporarily to at least 2 m below the surface or the ground level is raised to bring the platform suitably above the water table to allow for the dropping of weights.View chapterPurchase bookDynamic Compaction and Dynamic Consolidation of SoilsNathan Narendranathan, Eng Choy Lee, in Ground Improvement Case Histories, 201512.2.1 Deep dynamic compaction with drop weightsThe deep dynamic compaction (DDC) technique involves using a crane to drop weights of between 5 and 20 tons, from heights of up to 20 m. Figure 12.1 technique is best suited to large, open sites where few obstructions are present.Sign in to download full-size imageFigure 12.1. Example of deep dynamic compaction.Depending on the weight used and the drop height, the depth of treatment could vary from 8–20 m in sands. The number of drops, weights used, and the height of the drops depend on the required posttreatment bearing capacity, settlement performance, and soil conditions. Between two and five passes are generally required with the first or earlier high-energy treatment passes aimed at treating the deeper soil layer and a final low-energy contiguous tamping pass to compact the shallow near-surface soils disturbed during the earlier higher energy treatment passes. DDC is commonly used in reclaimed areas and landfill rehabilitation to provide a strong ground with less susceptibility for settlement or differential settlement.The authors’ experience has shown that DDC can be used in clays in combination with prefabricated vertical drains or jute drains that can withstand the impact of the drop weight.View chapterPurchase bookImproving Geotechnical Properties of Closed Landfills for Redevelopment Using Chemical Stabilization TechniquesBehnam Fatahi, ... Behzad Fatahi, in Ground Improvement Case Histories, 20158.3.1 Improvement techniquesThe deep dynamic compaction (DDC) technique is a common ground improvement technique because of its economical application. Zekkos and Flanagan (2011) reviewed 64 case histories with respect to the effectiveness of DDC on MSW landfill sites. With DDC, large voids are reduced, and subsequently other techniques such as fly ash–lime grouting can further decrease the remaining smaller voids. In addition, the lime/fly ash slurry injection has a significant impact on protecting groundwater, neutralizing leachate. Several research works have been published on the chemical stabilization of problematic soils incorporating some waste products such as carpet fibers (Fatahi et al., 2012, 2013a, b). Replacement of cement with by-product materials such as fly ash can further reduce the expenses associated with stabilization. Many researchers have reported the application of fly ash in geotechnical projects (Kawasaki et al., 1981; Kehew, 1995; Kitazume et al., 2000; Hokmabadi et al., 2014a, b). Horpibulsuk et al. (2012) reported that from an economic and environmental standpoint, rich materials in slaked lime (Ca(OH)2, calcium hydroxide) can be treated together with pozzolanic materials, such as fly ash, to develop a cementitious material. A series of laboratory permeability tests on decomposed MSW, using chemical agents, have been conducted to quantify the behavior of stabilized MSW samples.View chapterPurchase bookDeep Compaction of Granular Fills in a Land Reclamation Project by Dynamic and Vibratory Compaction TechniquesMyint Win Bo, ... Mahdi Miri Disfani, in Ground Improvement Case Histories, 20158.5 ConclusionThree deep compaction techniques—MRC, vibroflotation, and dynamic compaction—relevant for land reclamation projects have been discussed in this chapter. The following conclusions can be drawn:•All three techniques were found to be suitable for the densification of land reclamation fills.•The type of equipment, spacing of points, duration of compaction, and other operational considerations are of importance in the application of these techniques.•In the MRC technique, the selected frequency should be about the soil resonance frequency, and degree of compaction is found to be consistent with distance from the probe point.•In the vibroflotation technique, degree of compaction is found to decrease with distance from the probe point.•In dynamic compaction, the centroid point is found to be the most compacted point in the dynamic compaction technique, with the location directly beneath the pounder found to be the least compacted point.•An aging effect is significant for the vibroflotation technique, but it is not found to be significant for the other techniques. This can be attributed to the required injection of water or water jetting, which is applied in the vibroflotation technique, and the subsequent dissipation of pore water.View chapterPurchase bookDynamic Compaction and Dynamic Surcharging at Dubai’s Palm Jumeira Sewage Treatment PlantsBabak Hamidi, Serge Varaksin, in Ground Improvement Case Histories, 201510.3 ConclusionThis project demonstrated the effectiveness of combining dynamic surcharging and dynamic compaction for improving the ground and achieving results that would have been otherwise difficult, if not impossible, to achieve. Dynamic surcharging was able to induce additional settlements compared to what was realized under static loading conditions. This has not only shown the value of dynamic surcharging for increasing induced settlements and reducing soil porosity, but is also a reminder that even if settlements are acceptable under static loading conditions, vibration of the ground due to earthquakes or any other source can impose more settlement. In conclusion:1.Dynamic surcharging can be used to increase induced foundation settlement under static surcharge by 1.3–5 times, depending on the distance from the pounder impact point, to treat silty material that would normally not be treatable by dynamic compaction, and to increase the depth of treatment.2.Although the settlement magnitude of dynamic compaction was much more than dynamic surcharging, the latter induced critical settlement at depths that were treated less effectively with the allocated pounder.3.Excluding the highest values, average PLMand EM, respectively, ranged from approximately 2–4 MPa and from 23–30 MPa at depths of about 4–8 m. These values are significantly higher than what was suggested by Lukas (1986).4.Maximum improvement ratios were in the range of 10–18, which are significantly higher than the range that was suggested by Lukas (1986).5.Due to the combination of dynamic surcharging and pre-excavated dynamic compaction with a high number of blows, improvement can still be observed at greater depths.Fig. 10.23 shows Tank G-G after construction.Sign in to download full-size imageFigure 10.23. Tank G-G after construction.View chapterPurchase bookThe Changi East Reclamation Project in SingaporeMyint Win Bo, ... Victor Choa, in Ground Improvement Case Histories, 20159.4.3 Deep compaction of sand fillThree types of deep compaction methods were deployed to densify the granular soil: dynamic compaction, MRC, and vibroflotation. The dynamic compaction method was used in the area where the required depth of compaction was 5–7 m. The MRC and vibroflotation methods were adopted in the areas where the required thickness of compaction was 7–10 m.The effectiveness of dynamic compaction is dependent on the combination of weight, geometry of pounder, height of drop, spacing, number of drops, and total compactive energy applied. Experiments with different weight of pounder, height of drop, spacing, and number of drops were performed onsite to establish the most cost-effective combinations. The results of one of the experiments are shown in Fig. 9.18. The pounder used was 23 t. The height of drop was 25 m. The spacing between the pounding point was 7 m × 7 m. Only one pass of compaction was used. The number of drops per pass was 10. The total energy per point was 575 tons/m.Sign in to download full-size imageFigure 9.18. Cones resistance measured at various loctaions for 7m × 7m grid spacing (1) under the print, (2) intermediate point, (3) centroid point.After compaction, CPT tests were carried out at three locations, as indicated in Fig. 9.18. The CPT results obtained from the three locations are presented in Fig. 9.18. The variation of cone resistance with the location or the distance from the pounding point can be clearly seen. The cone resistance achieved at the center of the pounding grid (point 1) was greater and more uniform than that at the pounding point itself (point 3). The cone resistance achieved at point 2, which was between the pounding point and the center of the grid, was in-between the cone resistance obtained at those two points. Based on the experiments, four compaction methods, as detailed earlier in Table 9.3, were selected and used in the project. A comparison of CPT cone resistances obtained before and after compaction conducted at a number of locations using Method 1 (see Table 9.3) is presented in Fig. 9.19. The effectiveness of the dynamic compaction can be clearly seen in the figure.Sign in to download full-size imageFigure 9.19. Comparison of CPT cone resistance obtained before and after dynamic compaction at various locations.MRC does not require water for penetration. In this method, a steady-state vibrator was used to densify the soil. As a result of vibratory excitation, the friction between the soil particles is temporarily reduced. This facilitates rearrangement of particles, resulting in densification of the soil. A specially designed steel probe was attached to a vibrator, which had variable operating frequencies. The frequency was adjusted to the resonance frequency of the soil, resulting in strongly amplified ground vibrations and thereby achieving an efficient soil densification. Two types of MRC equipment and probes, as detailed in Table 9.4, were used. Experiments were carried out onsite to study the effect of the MRC compaction. CPTs were used to evaluate the compaction results.The results of one experiment using the MRC MS-200 H system are presented in Fig. 9.20. MRC tests were conducted at a square grid of 5 m × 5 m (see Fig. 9.20). CPT tests were conducted at five locations of an even spacing of 1.25 m in-between two MRC compaction points. The cone resistance versus depth profiles obtained at the five locations before and after compaction are shown in Fig. 9.20. It can be seen that substantial improvement was achieved at every point mainly within a depth of 4–10 m and the effect of densification does not appear to be significantly affected by the distance from the compacting points with the grid. The sand fill before compaction appears to have been mysteriously densified, possibly by seepage force or cementation at depths near 1 and 4.5 m, as indicated by the high CPT values at those two locations. However, this localized densification effect, if it was real, had been destroyed by MRC compaction. Nevertheless, as the sand fill was recently deposited, cementation or other effects would not be a consideration.Sign in to download full-size imageFigure 9.20. Variation of cone resistance with distance from the probe point after compaction.Several types of vibroflotation equipment, either electrically or hydraulically driven, were used in the project. The differences in the various types of vibroflotation equipment are shown in Table 9.5. Among the three types of vibroflotation equipment, the power rating is the same, but the centrifugal forces are different. The model numbers signify the amplitude. The amplitudes for V23, V28, and V32 are therefore 23, 28, and 32 mm, respectively. Keller S-300 type uses a higher power rating, but low centrifugal force and amplitude. Pennine type has a high centrifugal force and amplitude and its dimensions are all the largest of the three plant types. The spacings used for each piece of equipment to achieve the respective densification requirements are shown in Table 9.6. Onsite experiments were also conducted to study the effect of the vibroflotation compaction.The results of one study are shown in Fig. 9.21. Vibroflotation compaction using the V32 model at the 3.2 m triangle grid pattern shown in the figure was conducted. CPT tests were conducted at five locations evenly distributed between two compacting points. The cone resistance profiles obtained before and after compaction at each CPT test points are given in the figure. The results show that the effect of vibroflotation compaction is affected by the distance to the compacting point or the position in the compaction grid. The cone resistance is the highest at the probe point and the lowest near the center point of the triangle (CPT points 2 and 4). However, cone resistance at the center of the four compaction points (point 3) is higher than that at points 2 and 4. On the other hand, the improvement appears to be effective through the entire depth ranging from 1–10 m with more significant improvement felt at the bottom (see CPT profiles for points 1 and 5).Sign in to download full-size imageFigure 9.21. Variation of cone resistance with distance from the probe point after compaction.Each of the three compaction methods has its own advantages and disadvantages depending on the site and soil condition in the various areas. The dynamic compaction method produces a relatively more uniform degree of densification in each layer. However, the depth of densification is limited to 6 or 7 m. The MRC method is able to compact with a wider spacing and compact the sand at a relatively deeper depth. However, the probe needs to be maintained frequently as a result of wear and tear. The vibroflotation method can compact to a greater depth. The degree of densification is uniform along the depth, but can vary significantly with the distance from the probe points. It requires a smaller grid spacing and a sufficient supply of water.It has been reported by Mitchell (1986) that there is an ageing effect, that is, an increase in the CPT cone resistance with time, after densification in sand has been carried out. Such an ageing effect varies with the mode of compaction as far as the observations made in this project are concerned. As reported by Bo and Choa (2004), the ageing effect was the highest in vibroflotation-compacted sand. The ageing effect was not significant in sand compacted by dynamic compaction. One example is given in Fig. 9.22. The ageing effect due to MRC compaction is in-between that of vibroflotation and dynamic compaction.
- Home >
- Catalog >
- Life >
- Weight Chart >
- Wsdot Weight Table